Episode 5- A Legacy of Salts
Episode 5: A legacy of salts
�what we're seeing is there's, you know, the, the problem has sort of built up without us maybe being aware of it, because there's this huge lag time from when we put salt on the ground to when we're seeing it be a problem. I think we're really starting to just figure that out now, even though we we've known this has been a problem for decades.
I�m talking with Hilary Dugan, a researcher at the University of Wisconsin-Madison. For years, she�s been studying how chloride, mostly from road salt, has been slowly seeping into our lakes, rivers, and groundwater. I�ve been interested in this issue for years, for a couple of reasons. First, it reveals one of the many ways we�ve quietly but profoundly altered the world around us. And just as importantly, in order to understand the issue of nitrogen in the form of nitrate getting into water supplies, it helps to understand the story of chloride.
My name is Greg Klinger and I'm an agronomist and educator at the University of Minnesota Extension.� Together with my friend and colleague Shane Bugeja, I've spent the last few months interviewing experts in agronomy, biology, nutrient management, and ecology, trying to understand the story of nitrogen, in the hopes of explaining the phenomena we see out in the field, woods, and water.� Join us as we explore the different facets of this complex issue.
Episode 5: A Legacy of salts.
My name is Hilary Dugan and I�m an assistant professor at the Center for limnology at the University of Wisconsin Madison. So I'm a limnologist, we study inland waters, and I�m especially interested in water quality trends in lakes. I study a lot of lakes, here in Wisconsin�
�we see in the Midwest and also in the northeast US these sort of long term increases in chloride in a lot of lakes�
And so most chloride in the Midwest is from road salt. It�s the biggest contributor in most circumstances.
Can you walk me through how that chloride that's in that, you know, sodium chloride and the road salt moves from that road surface into, let's say, a nearby lake?
So when plow trucks puts down salts, usually put down solid rock salt, which is sodium chloride�
�it very quickly dissolves in water�
�once chloride is dissolved, it's going to go where the water goes. So if you're in a city that might be into the storm sewer, and it gets, it gets discharged into, usually a river or a lake. And so that's sort of a direct path- it's like the rock salt out goes onto the streets. As soon as it melts: storm sewer, river, and that could happen really quickly. Alternatively, some of that runoff might run off into a park or a lawn or something where you actually have water infiltrating soil, and when it does that, it gets into the shallow groundwater. And then, depending on your hydrogeology it can take paths that either go into deep groundwater or can again, discharge into a river, eventually, and those flowpaths can take different amounts of time. And so, you know, within a storm sewer example, your chloride�s going from your roads to your lake maybe in the same day, whereas if you're getting water going into your groundwater, that flow path might take months, years.
Why is chloride getting into water considered a problem worth studying? If you�ve ever seen pitted holes on the concrete floor of a garage or a rusted out car frame, a hallmark of life in the Upper Midwest, you know that chloride corrodes things: roads, bridges, cars, anything it touches. There�s evidence that high levels of chlorides running through water pipes can leach lead out of the pipe itself, an issue which got a lot of deserved attention recently during the Flint, Michigan, water crisis. It also creates biological winners and losers in bodies of water: high levels of chloride can stress fish populations, and can favor invasive species over native ones, like the notorious zebra mussels that can slice up your feet so bad when you�re out swimming in a lake or river.
Can you explain why chloride moves so easily in water?
Chloride is typically thought of as a conservative ion, which means that nothing really uses it. And so chloride is not involved in a lot of chemical reactions or biological reactions. So, unlike something like phosphorus, which we talk a lot about phosphorus because of water quality problems. But phosphorus is really in biological demand, like, algae want phosphorus. And so if you have phosphorus in a water column, you see it disappear. Whereas with Chloride, there's no organisms that really require chloride. And there's no chemical reactions that are taking it up out of the water column. And so any chloride that is dissolved in water tends to stay there. It also doesn't precipitate out very easily. So you don't, you don't get it sedimenting to the bottom of rivers or lakes. And that's the reason there's so much chloride in the ocean. So the ocean is almost entirely sodium and chloride and those two ions are there because nothing uses them...
And so once chloride is dissolved in water, it tends to stay there, and it'll just get flushed downstream. So it's possible to, you know, flush that chloride away, which is good for thinking about trying to lower concentrations. But you can kind of think of it having the same residence time as Just water, so it goes where water goes.
I'm kind of curious what you mean by that term residence time?
Yeah, so residence time is a term that limnologists like to use, and it means how long water stays somewhere. And so if we're talking about lakes, residence time refers to how long a molecule of water would stay in that lake before it was flushed downstream. So the bigger your Lake, the longer your residence time. So in a small lake that can be days, weeks, in sort of a moderate sized Lake, it's usually on the order of a few years. And then when you're getting, you're talking about something like Lake Superior, you know, you get up into hundreds of years. And so that really matters when you think about water quality problems. If you have a really long residence time, it's going to take a long time to fix that problem. It might also take a long time to get to be a problem�
Okay, so like, as an example here in Rochester, we have this dinky little reservoir on the main river through town that it, it's almost still like a river going through there, you know it, it slows down the water just briefly, but it's kind of still flowing. So if you had, let's say, no road salt for a long time, and then all of a sudden you put road salt down around there, that's gonna have a very quick turnaround and impacts versus something like Lake Superior. Is that kind of-
Absolutely. Yeah, and reservoirs definitely have shorter residence times. So they act more like rivers where, if we think about something like road salt, which is really seasonal, you'd have this big spike in chloride in the winter and spring and then it might come go back to baseline levels in the summer. Whereas a larger lake would kind of you'd have a more gradual increase in in those pollutants, for better or worse. So as opposed to having this acute shock to the system, it's sort of this gradual, chronic problem. It all depends on how long the water sticks around. And that's true of lakes. And it's also, you know, you can think of groundwater as the other end of the spectrum where your residence time can be hundreds to thousands of years, depending on your aquifer. And so once those pollutants are in your groundwater, you know, it takes a long time for them to get out.
Oftentimes, when someone discusses an environmental problem, they�d talk about different strategies to deal with it. There�s the prevention approach, stop producing the pollution to begin with; mitigation, which might be something like filtering chlorides out of our wastewater stream; adaptation- I don�t know in this scenario, stocking lakes with salt-tolerant strains of fish and microorganisms? How do we deal with the chloride issue?
In terms of environmental problems, this is like really, I'd say an easy one to fix. We deal with so many water problems that we might never solve and the chloride one is as simple as just stop, stop putting salt in the lake, stop putting it on our roads in the quantity that we do. And you know, we're starting to do that there's starting to be different mechanisms for just better road salting practices. So we're getting there slowly.
So is there any removal mechanism for chloride from the environment other than just washing downstream to the ocean?
Um, there's very little, okay, there's some retention in plants a little bit. They're going to take up water and that water has chloride and so a little bit of that chloride is going to get into the plant material�
But in comparison to other molecules or ions, chloride is by far one of the ones that really is not retained that much. So the mechanisms that do exist are are pretty low. I think this isn't really a mechanism retention, but soils just slow down that flow path and so you can have a lot of salt that's stored in soils. But you can think of it more as a reservoir where we've, we've put a ton of salt down on the landscape. And it's like, just haven't gotten to our rivers and lakes yet. It's just like still in the soil. But it has the potential to get there eventually.
Yeah, so it's just like a residence time issue in its own regard.
Yeah. And we refer to it a lot as sort of this reservoir effect, this legacy of salts that we just sort of haven't yet we haven't had to deal with yet because we're still waiting for it to show up.
Are there other chemicals that behave kind of similarly to chlorides in our water supplies?
�there's a lot of similarities between some of our nutrient runoff problems, nitrate being a big one in the Midwest...
�nitrate and chloride, you know, once they infiltrate into the soil, there's just so much of it that it's not being retained and it's just entering our aquifer. And it's, there are mechanisms for nitrate to, to break down into like nitrogen gas, but that doesn't happen in groundwater readily, and so we just have this sort of accumulation and build up that, you know, once it's in our groundwater takes a long time to get rid of. And so we start seeing, you know, problems with drinking water wells with nitrate and also with chloride. I mean, there's lots of cases where you have problems with both at the same time.
In the county where I live, there�s a bar that�s been getting its well-water analyzed every year for decades. When you look at how the chemistry of this wellwater has changed over time, you see a startling thing: about 20 years ago, the chloride levels in the well rose dramatically. Another chemical in the well began to rise at the same time: nitrate.
----
I think maybe we'll start with the sort of just the basics. So I was hoping you could just walk me through how water moves through the soil, and we can just start with a situation of rain falling on the ground, whether that's a field or a lawn or whatever. What happens to that water?
The first thing that we got to remember is we've got to get the water into the soil profile itself�
So, but if we kind of build a little scenario for ourselves to think about water getting into the soil,
you know, as raindrops fall on to the land surface, whether it's you know, got residue on it, whether it's bare and got crops growing on it, or, or whether we've got a lawn seeded, you know, we have to think about the pore space that's in that soil. So when we think about a soil, technically speaking, it's really composed of solids, which is the sand, the silt, the clay, and then pore spaces. And those pore spaces may be filled with air, or they may be filled with water. To grow a lawn to grow flowers to grow, you know, fruit trees, you know, or crops in a field, we need to have a good balance between the air portion and the water portion in those soil pores.
I�m talking with a colleague of mine at the University of Minnesota, Jeff Strock. Jeff is a researcher at one of the university�s research farms near Lamberton, in southwest Minnesota, who uses water management as a way to reduce losses of nitrate and other nutrients from farmland. Like chloride, nitrate has some negative effects when it moves through the environment. It doesn�t corrode pipes, but water with high levels of nitrate is considered unsafe for people or animals to drink, especially babies. And, as detailed in other episodes, it changes the biological winners and losers in different ecosystems, particularly in saltwater. Lastly, like chloride, it easily moves through the pores contained within the soil.
And so the soil pores once the water actually enters into the soil profile, are what actually move the water from one place to another. Now those soil pours when when we think of these, one really easy way to think about it is is that a soil pore network is kind of like a whole bunch of straws. And those straws are not one size of a straw. So you know, you go to your favorite fast food restaurant, and you get a straw, you can expect that every time you go back to that restaurant, you're going to get the same color straw, the same diameter. In a soil, you're going to get a large straw, you may get a little teeny tiny straw, you may get even a smaller straw, right. So there is a massive range of these different straws or different pore sizes that are in the soil. And they have different capacities to basically soak up the water.
This network of small, medium, and large straws will be different in every soil. The largest straws (generally you can see these pores with the naked eye) move almost all of the water through the soil after it rains. You�ll see a lot of these pores in sandy soils, or soils in a forest, or soils with lots of earthworms, and they�re what let these soils soak up water so quickly after a rainfall. The smaller straws, which can be smaller than the tiniest bacteria or virus, move water up, down, or sideways, but at a snail�s pace. You can see these pores in action when you water a houseplant through the saucer at the base of the pot, as they are what moves water up through the soil until a day or two later the top of the potting soil is now moist. In soils, these pores supply water to plants during the long stretches between rains. All sizes of straws will move water at least some of the time down towards what�s called the groundwater table- a layer low enough in the ground that all its pores are filled with water, making it a sort of underground sea within the soil. This groundwater table can be just a few feet below the land surface to tens of feet down or more, and if you�ve ever wondered how a creek can still have water running through it when it hasn�t rained for 3 or 4 weeks, it�s because water is seeping from this groundwater table into the creek, keeping it from running dry.
And then kind of following that to, let's say, going from the soil into a nearby stream, what's kind of the mechanism by which water moves through the soil to to a creek.
Right, so water movement from from the soil to some other water body, whether it's a lake, a stream, a ditch, for example, you know, that that's going to be governed by, you know, the landscape, where it's at, and what's basically underneath that soil. In some of the landscapes that we have in Minnesota, you know, say, the northern, maybe two thirds of the state, that there there is not artificial subsurface drainage, the water will get into that poor network. And eventually, the deeper that you go into the soil profile, you're basically eventually run into the shallow groundwater. Once that water gets to the groundwater, it's going to follow sort of the regional path- flow path- from the landscape to the receiving water body. So some of that water could go relatively deep, and then come back up into a lake or a river stream. Some of it might actually travel very short distances from a field or a forest, to the receiving Body. But basically, if you are in in, in a non-drained landscape, the waters gonna basically either take a short path or a longer path through the shallow groundwater to that river or that stream, that lake, whatever it might be.
How does drain tile in a field change that scenario?
So the drainage systems that we have today, really sort of interrupt that cycle to some degree of the water moving to the shallow groundwater, and then to a river or a stream. So the tile and there's short circuit, some of the water, not all of the water, but it's short circuit, some of that faster flowing water.
For those not familiar, tile drainage involves a system of connected pipes that are buried a few feet underground in crop fields and perforated with small holes. When the soil is wet, some of the water in the ground can move through these perforations into the pipe system, and from there water in the pipes usually flows out into a ditch or stream. But only some of the water, the water from the largest �soil straws� that Jeff described. Here�s why:
Imagine a sponge sitting in a bucket of soapy water you�re about to use to wash your car. When you pick up that sponge, water will come pouring out even if you�re not squeezing it, and over the course of a few minutes, that gush will slow to a steady drip. This water flowing out is called gravitational water, which simply means that it moves from the force of gravity alone, always downward. This is the type of water that flows through the soil and into a tile system, and it�s the equivalent of the water in large soil straws. But even when the water has stopped dripping from the sponge, it�s still plenty wet, full of water that won�t move under the force of gravity. You can only drain this water if you wring it out of the sponge or press the sponge against your car- it takes some pressure. This water does not get removed through a tile system- it stays in the ground, where it can either be used by plants or replenish the groundwater table. Functionally, a tile system shortens the residence time of that gravitational water in the ground, moving it rapidly out into the ditches and streams, when otherwise it would have moved more slowly through the ground towards those streams. For the farmer this brings the advantage of a soil that is sopping wet less frequently, allowing more days for fieldwork.
Drainage can lessen stress on the crop and often results in higher yields. It also makes it less likely that oxygen will be depleted in the ground, which would allow nitrogen burning microbes to remove some of the fertilizer that�s been applied. But it brings with it some water quality challenges. One of the main challenges is that chemicals that easily dissolve in water, which includes both chloride and nitrate, move along with it- they have a shorter residence time in the soil. And while this shorter residence time doesn�t impact the total amount of chloride loss that much, because as Hilary said, no living things really pull much chloride out of the ground, it can have a big impact on nitrate losses to water. Unlike chloride, plenty of things use nitrate: microbes breathe it so it disappears from the soil, plants pull it up into their root systems. The less time living things have to interact with that nitrate, the less of it they can remove.
The amount of nitrate leaching down through the soil, whether that�s through tile drains or towards groundwater that will more slowly feed the local streams and lakes, can be impacted by a number of factors. Two that can really impact nitrate losses are the amount of fertilizer nitrogen being applied to an area, as well as what specific plant is being grown. Whether it�s corn, potatoes, alfalfa, walnut trees, or turfgrass, characteristics of plants (like how deep or dense their root systems grow or what time of year they�re taking up lots of water and nutrients) can really impact how much nitrate the plants can remove from the ground. I wanted to ask Jeff about this.
So I feel like there's a general kind of continuum of thought about what's most important of the things that we sort of control to the amount of nitrate that gets lost. And some people would say that the most important things are what plants you're growing, essentially, irregardless of what amount of nitrogen fertilizer you're applying, other people would kind of make the argument that it's more about how much nitrogen fertilizer gets applied, you regard this somewhat unrelated to what you're growing? And I'm just kind of curious, what you what your thoughts are on that sort of kind of continuum?
Right. Well, you know, you make you make a really good point. You know, what I'm going to say from the experience that I've had from, what I've seen is, is that both scenarios that you just laid out are correct, right. So it does matter what you're growing. But it also can matter
what you're doing in terms of fertilizer application. I know that, you know, if we look at some of the data that we've collected, in lamberton, you know, some of our data in these data were collected from, you know, some small watershed scale size areas, so you know, 320 acre areas, two of them. One was was was basically, under conventional crop production, row crop production, the majority of it was corn and soybeans, it was fertilized, we measured the drain flow, the surface runoff. And then we had another adjacent to it, area. That was corn, about a third corn about a third soybeans, and then about a third small greens, but there was, and I'm giving some rough estimates here, right, because there, there were perennials on this landscape.
� At the end of the day, the one side where we had a more diversified cropping system, corn, soybean, small grains and perennials had lower concentrations of nutrients like nitrogen and phosphorus leaving that system and lower total loads of nutrients being lost from that system, compared to the one that was just corn and soybeans. So when you when you ask this question about, you know, is it the the cropping the choice of crops or choice of plants important? It really, really is. And it can be effective because even though we're growing corn and soybeans, where they require fertilizer, if we've got some other crops like small grains or perennials that are interspersed in there, that can have a dramatic impact on the water budget�
When he talks about a water budget, Jeff is referring to where water is going throughout the year. Water always seems to be relentlessly moving SOMEWHERE- when it falls from the sky as rain, it can infiltrate into the soil. Or if there aren�t enough large soil pores to take it in as fast as it�s falling, it can run over the land surface. From there it will either move through the ground in the directions of streams, ditches and lakes, or it will move upward as growing plants pull it up into their roots towards their leaves. But plants don�t use much water until they hit what I call their �teenage years�, when they�re growing rapidly and seemingly change overnight. Warm-season crops like corn or soybeans don�t hit that point until late June, early July in Minnesota; but cool season small grain crops like wheat or barley tend to hit that point much earlier, in May. And this ends up being really important to slowing the movement of water (and nitrate) to bodies of water, because in May, we tend to have a lot of gravitational water moving through those large soil straws towards tile drains and ditches; by July, in a normal weather year in Minnesota, plants have pulled most of the melted snow water and spring rainwater out of the soil. Most of the remaining water is in the small soil pores, and won�t really move into tile drains. So cool-season crops can have a large impact in reducing the amount of water and nitrate leaving the landscape. As Jeff mentioned, so can fertilizer:
So let's talk about nitrogen. So, by and large, the most important thing that that can help in terms of mitigating nutrient losses from agriculture, and specifically, but also from urban areas, in terms of nitrogen is the rate of the fertilizer application.
Take the example of growing corn in rotation with soybeans in the Midwest, something I�m far more familiar with than lawns. A typical rate of nitrogen fertilizer for corn grown after beans, depending on where in the Midwest you are, and what sort of soils your farm is on, might be around 150 lbs/acre. On average, the water moving down through the soil to a tile system or groundwater might have about 13 mg/Liter of nitrate in it. If a farmer increased their rate above that 150 lb/acre rate to 180 lbs/acre (a 20% increase), there�d be about a 31% increase in nitrate losses; if that rate increased by a third to 200 lbs/acre, now you�d have 62% more nitrate in that water. So, at higher rates of fertilizer, you can have a disproportionate amount of impact on the nitrate being lost from the field- crops just get less efficient at using fertilizer the more of it is out there.
On the flip side, dropping nitrogen rates farther and farther below that 150 lbs/acre rate has smaller and smaller impacts on nitrate losses. If you wanted to lose half as much nitrate from the field as that 150 lb/acre rate, research shows you�d have to drop your nitrogen rate down to about 0 pounds of nitrogen. That�s right- no nitrogen fertilizer at all. And here�s probably the main reason why: to get back to the analogy of the nitrogen conveyor belt from Episode 3 of this series, with no fertilizer, you have less of a crop being produced, which means less nitrogen demand by plant roots, but you still have nitrate being produced along that conveyor belt by soil microbes. In this case, the nitrate you�re seeing is coming from organic matter rather than fertilizer, but it can end up in water all the same.
we have to have a source of nutrient there to be able to move it right. So in southern Minnesota, and in fact, across the vast majority of the Midwest, when you get into sort of Iowa, you get into eastern South Dakota, Illinois, parts of Indiana, we have very rich soils, and those soils are relatively deep and dark in color. And that dark color is from decomposition of organic matter over a very, very long period of time�
� So in some of these soils, we have a natural storehouse of of nutrients. And nitrogen is one of those big ones that can come from these higher organic matter soils that we have�
These soils, even without a drop of fertilizer on them, can lose nutrients from them, just because anything that becomes dissolved in the water can move with it, whether you've added fertilizer.
And it�s important to realize there are negative consequences for growing nitrogen-demanding crops without any nitrogen fertilizer- first off, no nitrogen means some really quickly declining yields in a corn/soybean rotation. That is not particularly sustainable for the farmer, or for longterm grain production. And less yields mean less corn leaves, stalks, and roots, which means less organic matter being put back into the soil. Let�s go back to a question I asked Mike Castellano, a professor at Iowa State, who was interviewed in the previous episode. I was curious what would happen if year after year, you consistently applied MORE nitrogen fertilizer than you removed with your grain harvest, or you continuously applied LESS nitrogen than you removed in grain.
Yeah, so there's a few things to think about here...
�let's say we have a system where we're harvesting more nitrogen in the grain, plus the environmental losses, than we're putting in with fertilizer and legume fixation, right�.
�So if our outputs exceed our inputs, we will lose organic matter in the long run, we will lose carbon too, because that nitrogen has to come from somewhere and it's going to come from organic matter. We, however, cannot fix that problem by just putting in more inorganic fertilizer. Because as we run that nitrogen deficit over time, the carbon that was holding the nitrogen in the organic matter goes into the atmosphere. So there's no longer that carbon to hold that fertilizer in the field that we might want to offset that negative balance with. Instead the solution is to put more organic matter into the system to hold that nitrogen there. And so now this comes back around to why the fertilizer input that maximizes crop production is the best fertilizer input to maximize soil organic matter. And that's because if we don't put in enough fertilizer, like we said, to maximize crop productivity, we're not putting all the organic matter inputs back into the soil in the form of crop residue inputs. On the other hand, if we put excess nitrogen fertilizer into the system, there's no more crop residue inputs, right? Because we're putting in fertilizer that no longer increases the residue production, the crop production, there's no carbon to hold that nitrogen in the soil. And so it just gets washed out of the soil or it goes to the atmosphere and so it does us no good and it's just a loss of money to a farmer that pollutes the environment.
What would happen if you just shut off the spigot and stopped applying nitrogen fertilizer to your cropland for years and years?
The crop would have to start relying 100% on the microbes, the yield of the crop would be really bad. As the yield of the crop would go down�
�yeah, the losses will decrease, but the losses per unit of food production of that land will increase enormously, because the loss in crop production will exceed the improvement in environmental losses. By the decrease in environmental losses. As a result, you know, the nitrogen losses to the environment per bushel of corn will go up. And at the same time, we'll be mining our soil organic matter, which is completely unsustainable, right?
So, applying less nitrogen fertilizer will, all things being equal, mean less nitrate loss. However, applying less fertilizer to nitrogen-hungry crops like corn could have some pretty significant trade-offs below a certain level, when the crop is no longer getting enough nitrogen from organic matter and fertilizer to produce a lot of growth. By the way, we�ve been talking about a nitrogen fertilizer rate that�s too much, not enough, or just right to maximize your crop production as if it�s something we can know. Although there are research-based tools developed by land grant universities that serve as a great starting point for fertilizer decisions, the truth is, we can only know this �perfect� rate of nitrogen fertilizer after the fact. The challenge is the microbes in the soil that burn or breathe nitrogen or release it from soil organic matter control where nitrogen goes, not the plants. Because the activity of these microbes is mostly controlled by weather conditions, which change from day to day and year to year, that perfect nitrogen rate changes every year, even on the same farm growing the same crop. And often in ways that are unforeseeable.
�We are absolutely not in control of the weather. I remember a number of years ago, it was the last couple of weeks of September right before soybean harvest was going to kick in, in a 24 hour period, we had seven inches of rainfall. That was that was an astronomical amount of water. I mean, in the years I had been here in Minnesota, we had never had that much in a 24 hour period that I had ever witnessed�
�water is uncontrollable, the rainfall is uncontrollable, the actual timing of it, I mean, we just we can't do anything about that. And so we kind of have to live with it and design things for it. But you know, we can try to help manage some of that water.
----
A thunderhead builds and the sky darkens, with a curtain of rain visible on the horizon. A few large raindrops hit the ground with a splat, quickly joined by more and more, until the rain is thundering on the roof of your car, rivulets of muddy water are running over the fields, and puddles are forming in all the low spots of ground. The ditches and creeks start to swell over their banks within minutes as water runs off the roads and over the fields into them. If you were in a landscape where there was a lot of tile underlying the fields, the creeks might not rise as high initially, but over the next few hours the rain that had percolated into the ground and then moved down into the tile systems would be gushing out into the creeks. Along with that tide of water comes some nitrate that had been hanging in the pores between soil particles.
You can think of this water and the nitrate within it as moving along what�s often referred to as a �jerky conveyor belt�, a system that moves both from the land, through streams and lakes and rivers to, at least in the case of Minnesota, Hudson Bay to the north, the Gulf of Saint Lawrence to the east, or the Gulf of Mexico to the south. The conveyor sometimes moves the water along at a rapid pace, like when it�s moving through a tile pipe or down a river, and sometimes it grinds almost to a halt, like when it enters a wetland or is backed up behind a dam. Every time that water slows down there�s more opportunity for nitrate to be removed from it, for two reasons. First, the plants that filter some of the nitrate out of the water establish better in slow-moving water where their roots aren�t scoured away every time it rains. Second, slower water can lose its oxygen more easily, which allows nitrogen-breathing microbes to remove some of that nitrate from the water and send it back into the air. In a tile-drained landscape, where we�ve sped up this conveyor belt in the field in order to have better crop yields, better conditions for running farm equipment, and less nitrogen loss from the activity of nitrogen breathers, the solution is to find other places, outside the field, where we can do the opposite and slow that conveyor belt down.
At the University of Minnesota research farm in Lamberton, there is a research project looking at how a combination of different conservation practices, mostly involving slowing and treating water from fields, can reduce the amount of nitrate moving into lakes and rivers. A few fields on the farm get cover crops seeded every year, which helps pull some nitrate out of the ground and store it in vegetation until later in the season when it�s released back to the cash crop. Before leaving the farm, the tile water from some fields gets run through a bioreactor at the field edge, which usually looks like a chamber or trench filled with things like wood chips or corn cobs, where nitrogen breathing microbes remove some nitrate while growing on the plant material. The tile water from some other fields goes through small constructed wetlands before entering the ditch. Finally, in the ditch itself, there�s what�s called a low-grade weir (you can think of this as a very low dam, one you could easily step over) to slow the water down a little bit as it makes its way downstream.
The amount of nitrate in water flowing through the ditch is then measured and compared to the amount of nitrate in the neighboring ditch draining a similar amount of land that doesn�t have the cover crops or water treatment in place, so that researchers can see how effective this �stack� of conservation practices IN the field, at the EDGE of the field, and in the DITCH can be in reducing nitrate losses from fields.
Aside from how much nitrogen fertilizer gets applied on the landscape, what other things that you�ve researched at Lamberton can reduce nitrate losses to water?
�if we can increase cropping system diversity on the landscape, by either having, you know, pastures or these bio fuel crops, or just, you know, perennials in general, we can definitely see improvements in the water quality. Okay, I gave that one example where we had cropping system diversity, from our own experience, it vastly reduced the water flow and the nutrient losses from that landscape. Now, again, this can be kind of hard, because if farmers are out there, they're growing small grains, but there's no market for the small grains, or they're growing alfalfa, and we don't have enough livestock to be able to feed the alfalfa to, and we don't have another outlet for the alfalfa, why would they want to grow those crops? Right? So it's a little tricky when we know that there's probably some good solutions out there by increasing cropping system diversity, but we can't forget that, again, farming is a business and what we're asking the farmers to do, they need to be able to make money yet...
Okay and how well are the stacked conservation practices you�re studying able to reduce nitrate losses?
On average, we're thinking of about a 60 plus percent reduction in nitrogen removal. And that's after water has traveled through the soil profile in plots that have cover crops, it's flowing through our bioreactors it's flowing through our wetlands and then eventually to the ditch. It seems the more practices that we can put on the landscape, the better the water quality can be, short of being able to diversify the whole entire cropping systems.
we have an incremental increase in the impact on a nitrate for example, if we can get cover crops established Beyond Good fertilizer management for nitrogen, right. So fertilizer nitrogen management will get us so far, if we added cover crops, it would take us incrementally further down that line of improving the water quality from agriculture, for example�
The science that I've practiced and worked with tells me that, you know, if we can't have cropping system diversity out there, if we can have multiple practices in a watershed on a landscape implemented, that we can do a pretty good job of improving water quality.
Nitrate removal requires two things: a slowing down of water, and time. Diversity in plants growing across the landscape can help slow down water, because different plants use water at different times of year. Structures that hold water back in ditches and wetlands can also give plants and microbes time to remove nitrate from water. If you�re in the Midwest and can get all of your acres cover cropped, or run every bit of tile water through a bioreactor or wetland, you can get something like 30-50% reduction in the amount of nitrate leaving your farm. And if you can�t go all in on any one practice (it�s a pretty tall order), stacking several ones like at the Lamberton farm can get you to 60%. With that amount of reduction, just about any stream in the rural Midwest whose water is currently higher in nitrate than is considered safe to drink would become safe if these practices were done across the landscape.
Time is also critical for shedding light on water quality trends for things like chloride or nitrate, as in: HOW is the amount of nitrate or chloride in our local lake or river changing over time, and WHY is it changing the way it is? To answer these questions, you have to understand a concept called lag time, which means that a chemical showing up in a body of water today may not have been released to the environment today, or last week, or even this year- it could have been working its way through soil and rock and groundwater for a generation. Maybe longer.
-----------
In the late summer of 1945, a scientist named Julian Webb working for the Kodak film company began to investigate a weird complaint: clients were receiving X-ray film shipments where the film was already exposed, with strange black spots on it, as if it had been exposed to ionizing radiation. A month earlier, as part of the highly classified Manhattan Project, the military had secretly detonated its first large-scale nuclear bomb in the Nevada desert. The two events, as Webb would discover, were connected. For weeks, radioactive dust created from the nuclear explosion had been swirling around in the atmosphere, all across the world. Occasionally, some of the dust would encounter storm clouds, and drops of rain carried it down to earth in small quantities. Some of this rain-carried dust, coincidentally, made its way into a river whose water Kodak used to make the packaging its X-ray film was stored in, which caused some X-ray film to be pre-exposed when it made its way to the hospitals and dental offices where it was meant to be used. In tracing the source of the radiation ruining his X-ray film, Webb found evidence of a then- secret government program; he also inadvertently discovered a new way to determine the relative age of water, which is critical to understanding this idea of lag time.
Nuclear explosions created completely new radioactive isotopes never before seen in nature. And, these chemicals decay slowly at constant rates as they disappear over time. Because the vast majority of nuclear testing happened in the late 1940s and 1950s, these isotopes can be used as a marker of sorts. Imagine yourself conducting an experiment like this at your home: you go out, today, in 2021, grab a water sample from your drinking well, and send it off to a lab to test it for some of these radioactive chemicals. The test results come back with nothing. Let�s say you then came back in 10 years, took another sample, and this time there were high levels of these radioactive chemicals. Now you�d know your water was about 80 years old- there was about an 80 year time lag between when that radioactive dust fell on the land surface in the 1950s and when it finally seeped down into your well. You could also expect that if you took another sample 30 years later, the radioactive chemicals would be lower- a reflection of both the slowdown in nuclear testing after the 1950s, and the slow decay of the radioactive chemicals themselves. Scientists use these types of chemicals to get a rough age of drinking water, and that allows them to get a sense of when other chemicals have or will start showing up in drinking water. This age-dating has shown us that chloride and nitrate in water, at least at the high levels we sometimes see today, are due to the actions of modern humans- they DON�T show up in �old� water that was already in groundwater or that fell as rain BEFORE the nuclear bomb testing era.
The history of road salt thing is really interesting. I mean, it started pretty rapid, like rapidly in the 50s and 60s in the United States. And you we went from not salting you know, where's it wasn't a thing in the 40s to all of a sudden wanting to drive everywhere all the time. And a lot of states had clear road mandates where they said, you know, no matter what, we're going to keep the roads clear. And what that meant was dumping, you know, tons of salt per mile of road every winter.
Let�s get back to the well inside the bar where water analysis has shown chloride and nitrate levels started to rise about 20 years ago. It�s not that something changed 20 years ago to make these levels rise (and I should mention, while they�ve risen, it�s not to levels that are unsafe to drink, which is also true of the radioactive isotopes I mentioned before), it�s that something changed 60 years ago. Both the amount of salt applied to roads and the amount of nitrogen fertilizers applied across the landscape in Minnesota rose rapidly at that time- and it took 40 years for the rain that fell and carried chloride and nitrate with it to make it down to the layer of rock in the ground that the well was pumping from.
I reached out to an expert to explain this water movement through bedrock a little better, using a landscape we both know well- the Driftless area of southeastern Minnesota.
�My name is John Barry, I work for the Minnesota DNR as a hydrologist in the county groundwater atlas program, and we study groundwater in counties one county at a time��
�Can you explain how water moves into and through our layers of bedrock?�
�Sure. Um, to explain it to others, it might be best to think of a layer cake. So southeastern Minnesota is underlain by a large number of different types of sedimentary bedrock layers, and they range from carbonates which are like limestones and dolostones, to sandstones, but there are also shale layers, and they�re all stacked on top of each other, almost like a layer cake. And if you think of a cake, you can think of the icing on it being the soil layer in southeastern Minnesota. In some areas of southeastern Minnesota, there�s no soil layer at all, in others it�s very thin�
So, as water moves (precipitation comes down) water can move very rapidly through thin soil cover, there�s not much of it to kind of attenuate and slow the water down, and it moves into these sedimentary layers, and the upper layers are predominantly carbonates�
They actually have large fracture systems and conduits in them, so there are cave systems down there, sinkholes, springs, so water can move really quickly through these upper layers that have conduits and cracks in it. And once water gets to a shale layer, it can�t move vertically (downward) easily, but it can move laterally�
Where they�ll come back out as a spring or a seepage area, or to a stream, a trout stream or a river��
Our geology in southeastern Minnesota is a� layer cake (in my head, it�s a Neapolitan cake). Different layers have a quicker or slower ability to move water downward. So, in my imaginary Neapolitan-layer cake bedrock, where limestone or carbonate rock might be the chocolate layer, strawberry is shale, and vanilla is sandstone, water moves quickly down through the chocolate-limestone layer and seeps into a vanilla- sandstone (which coincidentally, is the type of water-holding rock or aquifer that almost all our drinking water comes from here). But when it hits that strawberry-shale layer, it slows down, and most of the water will seep out over the top of this layer, usually where comes to the land surface on the side of a hill. Most of this water ends up flowing out as springs that feed our streams. If you had more chocolate-limestone or vanilla-sandstone layers under that strawberry-shale, some water might seep over the edge of the shale and back into the other rock layers below. But it wouldn�t be a lot of water, and so the water that is contained in those deeper rock layers tends to be mostly really old, uninfluenced by what we�ve been doing on the land surface.
�Thinking about the water in shallow or deeper layers of rock, what�s kind of a rough age of the lag time- can you give an example of how long it could take water to move from the land surface down to different layers of rock?�
�In southeastern Minnesota, the St. Lawrence is one of the deeper shale layers, and water below the shale layer and above it typically has much different residence time. Residence time is the total time it took for water to get from the land surface where it came down as precipitation to when it�s discharged at a spring or a well or a stream, so we can look at water chemistry, and we can say that any water above the Saint Lawrence is hours to decades old�
When we use Carbon-14, we find that aquifers below the St. Lawrence are typically thousands of years old, so the Lone Rock aquifer would be thousands to about 5,000, 6,000 years old. But When you get into the deepest aquifer, the Mt. Simon, that water can be 40,000 years or more.�
�Wow.�
It�s hard to fathom, but if your drinking well is pulling water from the Mt. Simon aquifer, the water you�re drinking would have been percolating into the ground as rain or melting snow at a time when the land was a frigid tundra roamed by mastodons and saber-toothed tigers, devoid of people, road salt, and nitrogen fertilizers, excluding, of course, the occasional ball of mammoth manure.
The concept of lag time raises a couple important points. First, because it can take decades or more for what happens at the land surface to make its way down into the rock where a drinking well is located, or to the springs that feed the creeks that feed the Mississippi River that feeds the Gulf of Mexico, we have to be aware that the effects of our actions today might only become apparent during the lives of later generations. Second, because of lag time, in some places, water quality issues might not improve or might even get worse for years despite our best efforts today. For example, a spring gushing out of a mossy rock on a forested hillside whose water is on average 100 years old, has never really been influenced by the road salt that started getting put down on the blacktop road on top of the hill 60 years ago. If we decided tomorrow to stop using any road salt on that blacktop road, chloride levels in shallow, �young� groundwater just under the land surface would start dropping really quickly. But that �old�-water spring would see its chloride levels start to creep up over the next several decades regardless. The same is true of the nitrate coming from fertilizers and organic matter. And so if we want to understand how the salting practices of today�s plow trucks are affecting chloride levels in our lakes, or how our fertilizer management is affecting nitrate levels in our rivers or groundwater, we have to make sure the water we�re analyzing is young, influenced mostly by the last few years, like a layer of groundwater just below the land surface, or the end of a tile line. Otherwise, we can end up with a skewed perception of the consequences of our actions today, thinking things are better or worse than they really are.
---
I want to go back to the day this podcast series was first conceived, an evening spent along the Upper Mississippi River after a day of spreading fertilizer on fields. It�s hard to spend much time along the Mississippi River without being aware of how much the river�s character is defined by human actions. What once was a free-flowing river meandering through a broad, wooded floodplain is now a combination of wooded, braided river channels, open marshes, and large shallow lakes created by the 29 lock and dam structures built along the Mississippi from St. Paul to St. Louis.
The moment each one of those lock and dams were erected, the challenges we face in managing the river�s natural resources changed completely. Some creatures, like paddlefish, were harmed by the dams; some, like the canvasback ducks and tundra swans that stop here to rest during migration, benefited greatly. The river�s water has itself changed- the loss of the floodplain forests has lessened the river�s ability to absorb sediment and nutrients like nitrate during floods, while at the same time, the lock and dam structures capture more sediment within the open water pools of the Upper Mississippi. And, while it can be hard to connect all the dots, these changes in how the river handles sediment and nutrients have impacts on what organisms live within the river itself and the Gulf of Mexico it feeds.
Similarly, the reason this podcast came into existence was to connect dots- how in environments as diverse as forests and lakes, salty oceans and freshwater rivers, hay meadows and cornfields, nitrogen behaves both similarly and sometimes, surprisingly differently, with effects on what plants might grow in a forest, what microbes will grow in the soil, what plants or algae will prosper in a lake or ocean, or what weeds will thrive in a field. I came into this hoping to learn things that would make me a better agronomist, how we might control soil microbes to more efficiently use nitrogen fertilizers, how to know when a field might benefit from a lot of nitrogen fertilizer or very little. But I came out with something more.
One of the most powerful things about conducting the interviews we�ve done in this series is that you get to see the world through someone else�s eyes. A lake scientist, farmer, soil scientist, ecologist, or agronomist might all look at the same landscape of cropland, lakes, woods and streams, and key in on completely different things. And what they focus on teaches you more about the world you live in. When you see how the relative amounts of nitrogen, phosphorus, and silica coming into bodies of water like the Gulf of Mexico have changed over time, it�s easier to understand how that�s created biological winners and losers of different organisms floating in the water. When you see how the nitrogen we�ve added to the world have changed what plants and animals live all around us, whether that�s fertilizer added to a field or gases from a power plant raining down onto a mountain forest, you can understand the parallel line of changes underground, in the soil. Learning how dynamic nitrogen is in the soil, how it�s released and tied up by organic matter through the actions of microbes, can help you appreciate why the corn in one of your fields, or some of the plants in your garden, might be stunted and pale, or tall, robust and lush. You start to see the similarities and differences between chemicals like nitrate and chloride in terms of how they impact our infrastructure, environment, and health, how time can be a friend or an enemy in addressing those impacts. When you talk to a farmer, you get a sense of history, of how the land has changed during their tenure; and a feeling of connection to a particular piece of ground. Then you start to see the thumbprint of human activity everywhere in the world around us.
We live on an enriched earth, a world where nutrients like nitrogen and phosphorus are harnessed and move through our plants, soil and water faster than they ever have in human history. In this series, we�ve touched on some of the ways in which this enrichment has impacted the living things that exist in fields, streams, in soils and seas. But there is so much more to learn about the complex dynamics by which nitrogen connects every living thing on earth, from the smallest soil organisms to the tallest trees.
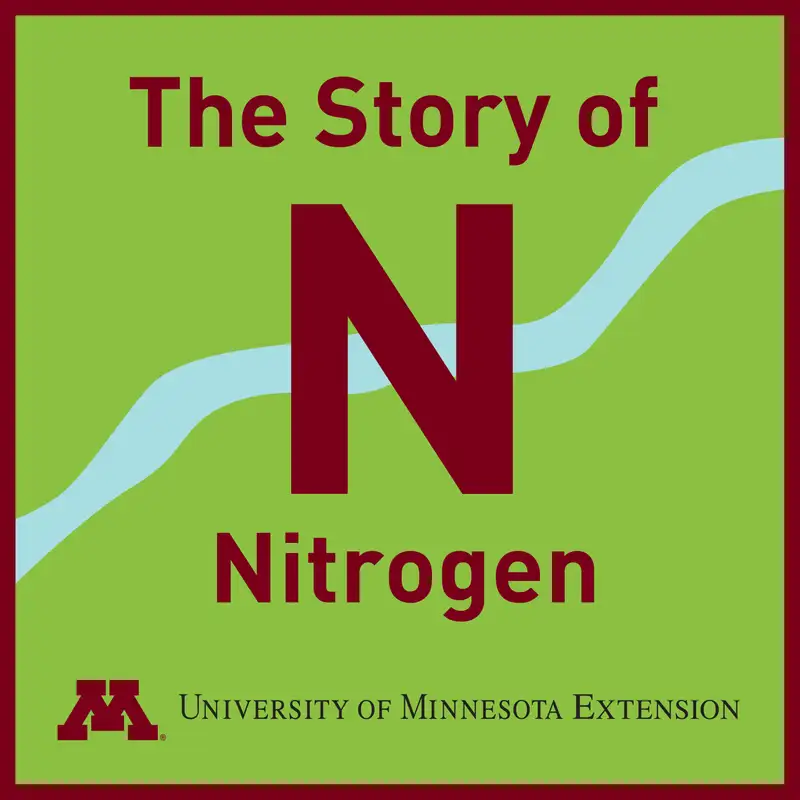