Episode 4- The Soil Bank Account
Episode 4: The Soil Bank Account
�And I think the main thing to keep in mind though is why do soils have high carbon? Right? soils have high carbon because they used to be cold and wet with short periods of high productivity in the summer, right. So basically what happened in these soils that have huge amounts of carbon like South Central Minnesota, North Central Iowa. This is what the environment was like before we came along and drained it: it was a huge wetland that dried out by July 1 with these enormous perennial grasses have huge amounts of growth for a few months, July through end of September, right. They produced a huge amount of biomass and then in the fall it got wet again. And that water didn't drain from the soil. And so essentially, it created like a big refrigerator for all that organic matter. And so you had these huge amounts of organic matter input, and then these environmental conditions have locked it away from being decomposed and released back to the atmosphere.�
My name is Greg Klinger and I'm an agronomist and educator at the University of Minnesota Extension.� Together with my friend and colleague Shane Bugeja, I've spent the last few months interviewing experts in agronomy, biology, nutrient management, and ecology, trying to understand the story of nitrogen, in the hopes of explaining the phenomena we see out in the field, woods, and water.� Join us as we explore the different facets of this complex issue.
Episode 4, the soil bank account.
Organic matter. It�s the black and brown stuff in dirt that lets it soak up water like a sponge, it�s what gives the air that fresh, earthy smell on winter days when the snow softens and melts into the thawing ground. It�s also the remains of the fallen leaves, plant roots, and dead organisms that live in the soil, and it�s the source of most of the nitrogen that sustains plants, and, by extension, you and me.
You can get a rough sense of how much organic matter there is in your soil just by digging, and looking at how dark the soil is, and how deep the layers of dark brown or black colored soil extend into the earth before the soil�s color starts to shift to tan, grey, red, orange, or white. Where I grew up in Virginia, if I were to go into my backyard, and start digging, first thing I�d find was a layer maybe an inch thick of oak leaves, ranging from completely intact leaves on top, to wet, small pieces of leaves stained black in the middle, to crumbly coffee ground-like pieces on bottom. If I started to dig below that, I�d find 6 or 8 inches of brown or tan dirt that crumbled into small clods, and below that, I�d rapidly find myself trying to dig through heavy, orange clay. Where the brown and black colors disappear, the organic matter levels are very low, and a soil without these dark colors at any depth probably has very little organic matter.
That range of material from whole leaves down to crumbly coffee grounds represents a continuum of organic matter, from freshly fallen down to older and wholly decomposed. The process of decomposition goes something like this: creatures like worms, ants, millipedes shred that fresh organic matter like plant leaves and stalks into smaller pieces, and smaller bugs in the soil further shred that organic matter. At the same time, tiny bacteria, fungi and other microorganisms are covering the surface of that shredded organic matter, exuding enzymes that soften and rot that material, finally breaking it down to its tiny, most basic molecular constituents. Some of this material can be directly used by plants and other living things for their own growth, and the rest will end up hidden within the structure of the soil, helping it hold nutrients and water and glueing together the pieces of sand, silt, and clay that make up the soil. Decomposition of organic matter is somewhat like deconstructing a brick house- the insects and earthworms are removing the mortar between the bricks; the microbes are dissolving and pulverizing the bricks back into the minerals they came from.
Here in the Upper Midwest, where our soils are young and fresh, the ground-down remnants of melted and wind-blown glaciers, and where the vegetation for thousands of years was prairie and open woods, I could dig down for several feet and still see black or dark brown soil, evidence of high levels of organic matter. We are blessed with rich, black earth and organic matter levels greater than almost anywhere else in the world. And it shows in our ability to grow crops that need lots of nutrients- because in addition to containing a lot of carbon, this organic matter contains- and releases for plant use- a lot of nitrogen and other nutrients. To learn more about this, I sat down with Mike Castellano, a professor at Iowa State University who thinks a lot about organic matter, and a lot about nitrogen.
�Mike, how much of the nitrogen a plant needs in order to grow- let�s say a crop like corn- can it get from the decomposition of soil organic matter?�
��generally, about two thirds of the nitrogen uptake by the crop comes from the soil organic matter. And roughly only a third comes from the fertilizer��
�-and that is largely because of those microbes, right? They're constantly eating and are spitting out inorganic nitrogen so fast relative to the amount of nitrogen that we put in the system and it kind of mixes it all up in a big soup.�
We think of fertilizers and nutrients in the soil as inactive, just sitting there waiting for plants to use them or to be flushed out of the soil under the right conditions. But, in reality, that�s not what�s happening. Fertilizer, nutrients, are far more dynamic. For example, as soon as a nitrogen fertilizer like urea has been applied and dissolved in the soil, there are microorganisms devouring it, using it for their own growth. But much like a small animal like a mouse reproduces much more quickly but has a much shorter lifespan than a large animal like an elephant, these tiny microbes tend to live and die fast, taking up and releasing that nitrogen over the course of just a few hours in many cases. Think of bread rising on the kitchen counter- in just a couple hours, the bread can double in size as yeasts eat its carbohydrates and pump out bubbles of carbon dioxide, until they run out of food, sputter out, and die. Microbes consuming the nitrogen from fertilizer can live, eat, and die on the same timescale.
To understand how powerful the influence of soil microbes is on nitrogen in the soil, if you picture a really good-looking corn field- something capable of producing 250 bushels an acre of grain- microbes in the soil are consuming as much nitrogen in a SINGLE DAY as all of that corn is consuming that whole year. On the flip side of the coin, though, they�re also RELEASING huge amounts of nitrogen every day, as wastes, or from breaking down organic matter, or when they die and are decomposed themselves by other soil microbes, and this released nitrogen, usually in forms like nitrate or ammonium, can feed plants.
These microbes serve as a sort of competitor with plants for the nitrogen in soils- most of our crops can only really take up the fertilizer forms of nitrogen like ammonium and nitrate- what Mike termed �inorganic� nitrogen- rather than the organic nitrogen forms found in dead microbes or soil organic matter. The patterns by which these microbes live and die over time controls how much of their organic nitrogen becomes available for crop growth at any moment in time and across an entire growing season.
---
�the amount of nitrogen fertilizer that the crop needs is a tricky balance in how much do you lose to nitrification? How much do you lose to leaching? but also how much are the microbes going to produce for you from the soil organic matter?
Picture yourself as an investment manager, standing in a glass sided building towering over downtown New York (at least that�s the image I have of an investment banker�) You�re responsible for managing millions of dollars in people�s money. At any given moment you�re going to have some cash on hand, but that money pales in comparison to the money that�s going in and out as you buy and sell stocks all day.
When I�m thinking about how much of the nitrogen in a crop field or my garden is available for plants to use, and whether I should have applied more or less fertilizer than I did, I always envision that nitrogen as money coming into or going out of a bank account- cash that plants can �spend� for their growth, but that they have to compete with microbes to get. But this bank account is more like the complex financial scenario of that investment manager, because relatively speaking, there�s always a lot more money tied up in assets (organic matter) than there is in cash (plant available nitrogen like ammonium and nitrate) and, like that investment manager, buying and selling huge amounts of stocks every day, far more nitrogen is being consumed and released by microbes every single day than is being used by plants, or even available to plants. Being able to predict how much �cash� will be available to a crop over the course of a growing season can help a farmer know how much nitrogen fertilizer their crop will benefit from, a goal that can help both farm economics and environmental quality. The challenge is how variable that can be: I�ve seen farms where the soil organic matter provides as little as 58 lbs/acre of nitrogen for the crop to as much as 244 lbs/acre in one growing season. With such a wide range, what controls how much nitrogen is coming into that bank account?
�it's the impact of our management on those microbes.
So management being things like what crop you�re growing, how much fertilizer we use, tillage, how we manage the dead plant stalks from last year�s crop?
�all these things are important, right. Some are more important than others, temperature and moisture will always be number one and number two.
In a single pinch of soil, there could easily be 10 billion individual bacteria, miles of fungi, and billions of other microbes like archaea, all living, breathing, reproducing, dying. Some of them grow best and reproduce fastest at cool temperatures, most grow best at warm to hot temperatures, and a few grow best at temperatures you�d more likely find in an oven than in the earth. And they all live within films of water that coat the particles in soil. While they can eat a wide array of different foods to survive, the majority of them live by devouring organic matter in the soil. In the course of doing this, they often release some nitrogen from where it�s locked up in organic matter, a process scientists call mineralization. Personally, I hate this term, because the image it gives me is of something crystallizing out of water like a stalagmite in a cave, lifeless and glacially slow, when the truth is that mineralization is rapid and intertwined with life in the soil- it�s the release of nitrogen INTO soil water where plants can access it with their roots. Regardless, because most microbes do best at warm to hot temperatures, and live within soil water, soil temperature and soil wetness are the two most important factors for how these microbial communities live, grow, reproduce, die- and release nitrogen.
Temperature is the number one factor affecting the microbial production of inorganic N and organic matter. You know, it's a microbial process, right? The response is exponential. So the amount of nitrogen mineralization increases exponentially with temperature.
How about looking at it from the the other side of the coin like the so you have more warmer conditions would be more breakdown decomposition. What about from like the loss perspective?
Well, yeah, yeah, you have more potential for loss then too, right...
Envision the Upper Midwest in late April, the last traces of the winter�s snows gone and the lakes free of ice. Swallows wheel and dive over the lakes, devouring hatching insects, and blackbirds nesting in the cattails dive-bomb your head if you get too close. Lawns are turning to vibrant green, trees are starting to leaf out, and most of the corn has been planted, as a lack of rain has let the fields dry out and warm up faster than usual. With soil temperatures hovering around 50 degrees, microbes are only sluggishly breaking down last year�s corn or soybean stalks, and only slowly releasing nitrogen from them. Like plants poking their heads out of the ground in nearby woods, microbes� activity is slow, tentative. But as temperatures creep up over the next few weeks under the bright sun, it speeds up. The amount of organic matter they�re decomposing when the soil hits 60 degrees is twice as much as at 50 degrees; and it doubles again by 70 degrees, by 80 degrees, and so on, so that if the microbes were releasing, say � a lb of nitrogen per acre per day from organic matter at 50 degrees, at 60 it would be 1 lb per day, 2 lbs at 70, 4 lbs at 80 degrees. But this only happens if the soil stays moist. If the soil starts to inch into drought territory, all the films of water that coat the sand, silt, clay, and organic matter particles in the soil start to shrink until they only cling to the smallest gaps in the soil. And microbes begin to shrivel up and shut down, too, as the soil water that serves as their home disappears. The breakdown and release of nitrogen from organic matter grinds to a halt. If, on the other hand, the soil stays moist, microbes chug along, breaking down the dead plants and other organic matter that is their food, mineralizing huge amounts of nitrogen for plants to use, especially as the soil gets really warm in July and August. A lot more income is coming into the soil bank account, but there�s also the potential for more losses from that account as well- IF the soil gets really sopping wet for days after it�s already pretty warm, because then nitrate produced by nitrogen burners can leach down through the soil, and nitrogen breathers can exhale that nitrate back into the atmosphere. Mike used the analogy of soil as a sponge to explain the delicate balance of nitrogen inputs from organic matter vs. losses of nitrogen when the soil is warm and moist and microbes are very active:
�They're going to be pumping out all this nitrate in the soil, but it's not going to be lost, really, because the soil is at the perfect water holding capacity. Right? Think about that sponge that you take out of a bucket and you squeeze it Or you just let it drain on its own right? And it's not losing water but still pretty wet, right? And then let's say so they're producing all this nitrate and then you get a big rainstorm it saturates the sponge and boom, all gets leached out. Right? All that nitrate gets leached out�
If you want to measure how nitrogen available in the soil bank account is impacted by the weather, you have to realize that nitrogen coming into the soil from organic matter is an endurance race (impacts of the WHOLE growing season�s weather matter most), but nitrogen being lost is a sprint (a few days with the wrong weather can make a big difference). And we see this in research trials all the time. In Minnesota and Iowa, the years where cornfields need the least amount of nitrogen to produce good-yielding crops are warm years- as long as they don�t get too dry or way too wet. The years where crops need a lot of nitrogen to produce a crop, and yields are generally not very good, are when the spring is really wet, and the summer is dry. Under these conditions, losses are high early, and the dry summer weather prevents nitrogen from being mineralized when the crop is most dependent on it.
Although we can react to it, we can�t control the weather. But, as Mike alluded to earlier, we can manage our soils in such a way that the climate WITHIN the soil is altered, and this will affect the soil bank account of nitrogen. Think of what can happen just by switching from a common, two-pass tillage system to a strict no-tillage system. To those who might not be familiar with what soils under these systems might look like, imagine walking across a farm field under the warm sun of some dewy May morning. If that field were a no-till field, a majority of the time, your feet would be crunching on the light tan remains of last year�s corn stalks and leaves as you walked. Maybe only one-third of the steps you took would be on the chocolatey-brown dirt. If that same field were under a two-pass tillage system, where the soil is broken up into large clods in the fall and then lightly mixed, smoothed and evened out in the spring, the situation would probably be reversed- because a significant amount of corn stalks would have been mixed into the soil by tillage, as you were walking across the field, probably 2/3 of the time you�d be walking on brown earth, and 1/3 of the time you�d be walking on corn stalks. This would translate into a number of changes that will affect how much nitrogen is available to that year�s crop. First, that chocolate brown earth, being darker, will absorb more heat from the sun than the tan cornstalks, and so the tilled field is going to warm up and dry out quicker in the spring, and generally just stay hotter and drier during the growing season than the no-till field with more cornstalks on top. I asked Mike what some of the impacts would be on the soil bank account of nitrogen based on whether a field was tilled or not:
You know, I think that's like- it's a tough question and too general because the reality is in some years, it'll affect it one way and some years ago affect another assuming we're comparing it to a tilled, right? In general, on average, no till results in a cooler, wetter soil, at least in the initial years after beginning no-till. And, and in some places that may enhance decomposition because let's say in some places, water may be limiting decomposition whereas temperature is not. In other places, let's think southern Minnesota and Northern Iowa, where a lack of temperature and too much water are limiting decomposition generally, no-till will slow that decomposition process, which means there will be less nitrogen available for the crops to take up. Now, that said, it's a really complicated thing and that's why I hate to make generalizations about this because over a few years, you know you may get some return of macrofauna like earthworms, that despite you know, not tilling the soil, you can get some natural aeration of it and that can minimize that impact on wetness and temperature, things like that. So it's a really complex question that doesn't have a single answer, particularly due to year to year variability, you know, and really the management variability from field to field.
Speaking of that year-to-year variability. You mentioned that too much water or too little could sometimes limit the release of nitrogen from organic matter. Could you explain that a bit more?
�I think a good place to think about that would be like the prairie pothole example. Right. So prairie potholes, you know, water�s limiting you one year, let's say it's a dry year like it is in Iowa this year, your highest productive areas, the areas where the microbes are spitting out the most inorganic nitrogen, are going to be in the bottom of those potholes. Because water eliminates them in the rest of the landscape. And they're virtually doing nothing on the knobs and on the higher areas. Whereas in a wet year, it's going to be the total opposite. They're going to be inundated, drowned out by water in the bottom of the pot-hole, they're not gonna be mineralizing much at all. In those areas of the landscape that can dry out despite the wet conditions, they�re really pumping it out.
There are so many other cascading effects that impact the inputs and outputs of the soil bank account of nitrogen depending on whether and how aggressively it�s being tilled. How does that tillage impact the amount of compaction in the soil? As discussed in the previous episode, more compaction could mean more loss of nitrogen from nitrogen breathing. There�s evidence from studies in North Dakota that nitrogen fixing bacteria living in the soil add more nitrogen into the soil under long term no-till management. That�s a win for no-till agriculture�s ability to add more money into the soil bank account. At the same time, if the extra cornstalks on the soil surface keep the soil wetter, you could have more nitrogen breathing, and be losing more money from the soil bank account. Unless, of course, the lack of tillage allows more earthworms to colonize the field, chewing up and moving some of those cornstalks down into the soil while carving more channels through the earth, which allows the soil to drain water better and warm up faster. The potential impacts are almost endless.
Beyond soil temperature and moisture, another factor that has a big impact on how much nitrogen comes into or gets pulled out of the soil bank account is what happens to the remains of the previous year�s crop. Across the Upper Midwest, if you were to be out walking fields in early April, you�d generally see one of two things: the chopped up remains of last year�s soybean crop, which would look like small pieces of crunchy grayish mulch, or the tannish segments of last year�s corn stalks and papery remains of corn leaves. If you were to keep walking those fields over the next couple months, as the new year�s crop started to push out of the ground and stretch itself out, you�d see that soybean mulch disappear before your eyes, leaving behind the dark brown of bare earth, while the cornstalks would mostly stick around. And the differences in how those crops decompose translates to differences in how they release nitrogen. That field with decomposing soybean mulch- also often called residue, stover, stubble or trash- would typically be expected to release more nitrogen from organic matter, and thus require less nitrogen fertilizer for its crop, than the field with decomposing cornstalks. There are a number of theories that have been used to explain this: the first is that because soybean is a legume that fixes nitrogen it would add more nitrogen into the soil bank account while it was alive and growing, and this added nitrogen would carry over to the next crop. The second school of thought is that because soybeans are legumes, their tissues are more concentrated with nitrogen than corn, a grass. This means that as microbes are decomposing that soybean residue, they�re getting enough nitrogen from the dead plants as they need to live off of the carbon the soybean residue contains, and as a result they don�t pull nitrogen out of the soil to break down the soybean residue, while with corn residue, they do pull nitrogen out of the soil, resulting in less available for the next crop. This is the �carbon-to-nitrogen ratio� theory, which says that any dead plant matter that has only a small amount of nitrogen in it relative to the amount of carbon in it (which is what plant-decomposing microbes rely on for fuel) will always tie up nitrogen in the soil as it decomposes. There�s a third theory, which says that nitrogen fixation and nitrogen concentration don�t matter much at all, and that it�s really the impact of that dead plant material on the physical environment in the soil that�s important. I asked Mike about why crops need less fertilizer in the year after soybeans or other legume plants are grown, which, going back to the bank account analogy, is often called a �nitrogen credit�:
I don't think the term should be used. It's a really bad term. You know, I assume you're referring to nitrogen credits from legumes. The problem is nobody's ever looked at, you know, credit in terms of a balance system. So, this credit thing comes back to the fact that you can put in, you can grow a corn crop following soybeans with, you know, 30% less nitrogen and you'll get 15% greater yield. So it's a win win situation, right, versus, I should say, versus continuous corn, right�
�And people have attributed this lower requirement for nitrogen to the fact that the previous crop was a legume, so it must have put that nitrogen in the system. Well, our research clearly shows that that's not the case. The legume soybeans actually result in a lot more nitrogen harvested in grain than they fix from the atmosphere. You know, it's really underappreciated. So there's about three and a quarter pounds of nitrogen per bushel soybean, right. So like we take about a 60 bushel an acre harvest for soybeans, you know, we're looking at 180 pounds of nitrogen, huge amounts of nitrogen removed in the grain. And so it's not a credit. Yeah, you do have to put less nitrogen following soybean crop than a corn crop. So where does that come from? It comes from better conditions for the microbes to mineralize soil organic matter following soybeans than following corn. And a really good piece of evidence to clearly demonstrate this is let's say that continuous corn system and go back to your residue harvest example. If we harvest 100% of the corn stover along with the grain, we get a system that in terms of yield and fertilizer requirement looks very, very much like the corn following soybean�
Then I guess it�s more a nitrogen fertilizer penalty growing continuous corn with all its residue than it is a credit for growing soybeans.
So with this continuous corn penalty-
Yeah, yeah.
So you would say it's largely related to microbes and and the environment that is being created for them.
Right, yeah, the environment that's being created. We make the soil a lot warmer, we allow it to dry out faster, conditions for nitrogen mineralization are much improved.
What about the carbon-to-nitrogen ratio of cornstalks vs. soybean stubble? Does the lower ratio in soybeans have an impact on that nitrogen credit?
Yeah, I think it's important for sure.
I still think, using the corn soybean example that the effect of less residue on the soil temperature and moisture are more important than that ratio of the crop residue.
So how much nitrogen there is in the dead plant material, relative to its carbon content, matters to whether it pushes the soil bank account more towards tie up of nitrogen in microbes as they decompose the plant material, or release of nitrogen from the plant material that the microbes don�t need. But also importance is how that plant material breaks down, which impacts soil temperatures and soil moisture- too much residue can slow the release of nitrogen in wet, cool years or wet cool climates, while too little can slow the release in dry years or dry climates.
And there are other situations in nature where changes in how fast leaves and dead plants break down, and the subsequent impact on how warm and dry a soil is, can change the balance of the soil bank account of nitrogen. One of the best examples of this comes from observing the changes in our forests that have come about through the introduction of a foreign invader- an animal that traveled with colonists from Europe to North America- the earthworm.
�So when a gardener looks at an earthworm, generally they see- and a farmer- they see a sign of productive, healthy soil. You know, when a when an angler looks at an earthworm, usually they see something that they can catch walleye with. When you look at earthworms, how do you view them?�
�Well, I view them as having a lot of different roles in a lot of places. In cornfields, they're a good thing, in forests, they're a very bad thing. They threaten the conservation of many species of native plants in Forests and even the health of the whole forest ecosystem�
But you know, they're just doing what they evolved to do and we've moved them into a new continent .�
I�m talking to Lee Frelich, a professor and researcher at the University of Minnesota. One of his research interests for years has been how the introduction of nonnative earthworms change the forests of Minnesota.
If you were to travel back in time 20,000 years ago, most of Minnesota was covered with a 2 mile thick ice sheet that ground most of the earth down to bare rock, churning up rock fragments into the ice itself. In southeastern Minnesota, which the most recent glaciers did not cover, the landscape was probably a frigid open tundra of grasses, low shrubs, and lichens, with permanently frozen layers underground.
Neither of these environments are very hospitable to earthworms, and so as the glaciers retreated and disappeared, the landscapes that emerged had no earthworms in them. They didn�t have much in the way of trees either, but trees, because their seeds can be spread by the wind, or by passing through the guts of animals that travel long distances, recolonized Minnesota and the rest of the Midwest fairly quickly. Earthworms, which aren�t as good as seeds at surviving a trip through something�s gut, don�t migrate that fast- maybe only 15-20 feet a year. Or, to put it in other terms, they would have migrated about 35 miles total since the glaciers disappeared.
As a result, the forests that developed in Minnesota lacked any sort of earthworms until European colonists arrived. The organic matter in these forests grew into thick layers, and most of the �income� to the soil bank account of nitrogen in these forests was happening aboveground, in the layers of decomposing leaves. Now, with huge numbers of earthworms, those leaves are being mixed with the soil and that income is coming into the soil bank account much faster.
�We have some parts of the world where the forests were free of earthworms. And that was true for the lake states area: Wisconsin, Michigan and Minnesota, with some limited exceptions. There are a few native worms in southern Michigan and Wisconsin, but basically we have an area that was earthworm-free since the last glaciation. And now the European earthworms have invaded and now the Asian earthworms are coming in on top of that, so it's an opportunity to, to look at this gigantic landscape scale experiment that these worms are carrying out, I guess.�
�Lee, how specifically do earthworms change a forest?�
�They eat the forest floor, which is what most people call the Duff, it's leaf litter. There's many years accumulation of leaf litter in these earthworm-free forests. Plants are actually rooted in that leaf letter which can be up to 100 years old and at the bottom of the leaf litter, it's broken down into little fragments and even into black humus a lot of the plants and tree seedlings are rooted in that. When the earthworms invade, they eat that and they literally eat the rooting medium out from under most of the plants�
So in an earthworm free forest you have litter, which is thick, it's spongy when you walk on it. It's like walking on a memory foam mattress. It's very flat, you know and the leaves are all stuck together and bound together. It's almost like a piece of carpet that's soaking wet or a thick piece of felt. And with the earthworms that is just simply gone
also the soil becomes warmer in summer because that leaf litter would insulate the soil and keep it cool just like mulch does in a garden so you have soils that are warmer, drier...
And some of the key nutrients like nitrogen and phosphorus, potassium and calcium are leached out of the soil under these new conditions, so it becomes warmer, drier and more nutrient poor. And that has huge implications for the forest...
�So Lee, what are some of those implications?�
In sugar maple forest, which is the most common forest type from New England all the way out to Minnesota �
trees actually grow about 30% less based on our measurements.
So we have hundreds of native plants that grow in these forests and a lot of them are reduced hugely in abundance when the earthworms invade. They just don't like the new conditions .�
�If I were out walking in the woods kind of near my house or something, other than digging up the soil and looking for them, how could I visually tell whether I had earthworms or not?�
�Well, if the European earthworms are there, by midsummer you'll have patches of bare soil throwing showing through because the, you know, the leaves will be gone. The surface of that soil will be quite hard if it hasn't rained in a few days. And it will be far from this thick spongy layer of leaf litter that you would have had before�
And you'll see leaves that are skeletonized where they, they essentially eat the leaf by section and so they vacuum the cells out between the veins of the leaf and sometimes you'll find a leaf that you can unroll it and the entire skeleton of the leaf all the veins are there and all but all the tissue is gone. So those are all signs of earthworms. And of course, if you go out there in April on the first night that's warm enough, and if it's been raining and the leaves are wet, if you go out at night, the forest will be incredibly noisy. And that's from the nightcrawlers moving around under the leaf litter finding leaves they want to eat and dragging those leaves back to their burrow, and if you have 100,000 nightcrawlers per acre, and they're all out there, say, at midnight on the first warm rainy night which is usually like late April, you have 100,000 worms dragging leaves through all the other leaves on the forest floor it it can be amazingly noisy.�
�so you also said that the soils become poor in nutrients and kind of lose some through leaching. Could you go into a little more detail on how that works?�
�Sure, yeah. So the worms eat the leaves. And the decomposition happens inside the worms. So, an entire year�s leaf litter can be eaten in a few weeks if you have a cool wet period, you know, because worms can only eat the leaves when they're wet, of course, because otherwise they wouldn't be able to process them metabolically. You know, for an earthworm to eat an oak leaf that's dry would be like you or me sitting down and eating 10 or 20 pounds of flour, you know, it just just isn't going to work. But if you get a rainy cool period they can eat many of the leaves in October�
or they can do it in May, and in either case the trees are dormant. So all those nutrients are released because the worms break them down.�
�These nonnative earthworms decompose all the fallen leaves and dead plants, and that releases nutrients.
Do you have more decomposition overall with earthworms? Or is it just that the timing of decomposition is focused in you know, like, like you said, October and May and less at other times a year?�
�Yeah, it's a it's a much faster rate of decomposition, as a typical leaf would sit on the ground for 10 or 20 years before it's completely decayed before the earthworm invasion, and now, essentially, in less than a year, they're completely decayed. And that's because they're eaten by the earthworms, and they're decaying inside the earthworm, which is basically just a tube filled with microbes. So, yeah, it's a huge speed up of the rate of decay. And that's why the nutrients are released faster than the trees can take them up. And that's why nutrients are lost to the ecosystem.�
Income to the soil bank account of nitrogen is speeding up in these forests, 10 or 20 times faster than it used to be, both because worms are breaking that leaf litter down faster, and because by removing it from the soil�s surface, much like tilling corn stalks under in a field, they�re creating an environment that is warmer and drier and more conducive to quick breakdown of organic matter within the soil itself. If you remember the interview with Mary Beth Adams from Episode 2, some plants in the forest can adapt to this change, at least somewhat, others don�t. A forest is poorly suited to have fast release of nutrients when trees are dormant, because, while something like corn gets those nutrients added back by the person who manages that crop every year, or every few years, a forest is dependent on efficiently recycling its nutrients. When the nutrients are gone, they�re gone.
So if we think of these earthworms as having negative effects on our forests, why do we think of them as so positive in a farm field or garden? What�s the difference?
�In areas that are infested with worms, or where worms are native, the, ya know, the whole mass of the, especially the mineral soil is the castings of worms. So when the European worms invade, their castings are very dense, they have a high bulk density and they pack together quite well. So you actually have a rise in the bulk density of the mineral soil. It becomes quite hard and quite dense. So that is a big change in the structure, and that causes more overland flow of water during heavy rain events because it doesn't soak in as fast�
You have to remember before the worms invade you have a thick leaf litter layer, the rain can go in the leaf litter, and then slowly go from the leaf litter into the soil. So that's gone�
Now in cornfields, they can enhance the infiltration of water�
because the water can go down the burrows and it's going to go down two or three feet so it can go down and give water to the deeper roots. And cornfields have very hard soil. So it's not surprising that that worms would actually lower the bulk density there a little bit and cause more infiltration, but in a forest you naturally have a very spongy soil and the European worms actually increase the bulk density and reduce infiltration of rain.�
When Lee uses the term bulk density, he�s mostly talking about how compacted the soil is. A compacted soil can�t take as much water in, which is a good thing next to a house foundation, when you don�t want water to get in your basement, or around a landfill, where you don�t want pollution to leak out, but is not good for plants.
When he says worms increase the bulk density in a Minnesota forest, and decrease it in a farm field, what he�s describing is not an improvement in the soil from worms; it�s a convergence. Soils like those in a forest are being compacted and losing their ability to soak in water as fast; soils like those in a field, where there isn�t a spongy layer of residue on the soil surface, can soak in more water as worms loosen the ground. Whether worms are good or bad really depends on where you�re standing.
------
�We only have crops that have nitrogen demand in excess of the microbial production from the organic matter for, I don't know, like, 70-80 days a year. That's it. Right. That's, that's the problem. So these microbes are producing inorganic nitrogen from the organic matter whether the crop is growing there or not�
If there's no crop there to use it- I mean, the microbes aren't creating nitrate as a community service to farmers, right? for corn crops,. They're doing it as a byproduct of their metabolism. And if it's warm and wet, they don't care whether corn, soybeans are growing or not, they're doing it right?�
I�m talking again to Mike Castellano, about strategies to better match plant demand for nitrogen with its supply in our cropland. Corn and soybeans- our dominant crops- grow when conditions are warm. And when growing these crops, a similar situation arises to what happens when earthworms come into a forest that evolved without them. Organic matter is broken down and nutrients released when nothing is there to use them, but for an even longer part of the year than in the forest.
To combat this- to keep the soil bank account balanced, and nutrients in place- many people, from scientists to farmers to conservationists, advocate for more diversity in cropping systems. This comes in two different forms: first, by having less land devoted to warm-season crops like corn and soybeans and more devoted to cool season crops like oats or alfalfa; second, by keeping the warm-season crops in place, but squeezing in a second, cool-season crop before or after them, to catch nutrients that would otherwise be lost. This is known as a cover crop.
We�ll talk a little bit about cool season crops like small grains or forages in the next episode. Cover crops, though, are worth talking about in detail here, both because they can help balance the soil bank account of nitrogen, creating demand for the supply of nitrogen being released from organic matter in the fall and spring, and because how they�re managed can really impact how much plant available nitrogen (cash) will be there from the dead and decomposing cover crop for the next crop, like corn or soybeans, to use.
---
In you were to compress into a single 24 hour day the growth of a grass cover crop like wheat or rye, from when it emerged from its seed until it died after flowering and producing its own seed, for a long time (about the first third of the day), it wouldn�t be growing a whole lot that we could observe. Each plant would form a number of stems so that it covered more of the ground surface, but it would stay short and compact. Then, over the next third, the plant would just explode in growth- stretching taller so fast you could see it growing as you watched. In the last third, the plant wouldn�t get much larger, but it WOULD flower, produce its grain, and then slowly turn golden-brown as the grain ripened and the plant died. And these different stages have a huge impact on how much nitrogen will be available for the next crop.
During that first 8 hours of the grasses condensed 24 hour lifecycle, it�s not putting on a lot of growth. It�s still establishing a root system, and it�s still relying a lot on the nitrogen that was packed into the seed it grew from, so there�s not a lot of nitrogen uptake happening from the soil. If your goal is to balance the soil bank account by increasing plant uptake of nitrogen being released by microbes, a crop at this stage just won�t do much.
In the next 8 hours of its lifecycle, though, that plant is going to take up a LOT of nitrogen, as its stems elongate and reach upwards towards the sun. A cover crop in this stage of development can do a tremendous amount to balance the soil bank account. In the last 8 hours of its condensed lifecycle, that wheat or rye plant takes up little nitrogen from the soil; instead it just moves nitrogen from its stems and leaves into its flowers and grain. But it�s still photosynthesizing, still accumulating carbon. So a plant at this stage of growth isn�t balancing the soil bank account any more effectively then if it were in the previous stage of rapid growth. Something else is happening as well- as its stems and leaves are losing nitrogen to grain but still accumulating carbon from photosynthesis, the carbon-to-nitrogen ratio of the plant increases. You can think of the effects of a cover crop killed after flowering versus one killed during its rapid growth stage as comparable to the effects of corn versus soybean residue- like the corn residue, the post-flowering cover crop will tie up more nitrogen in the late spring and early summer when it�s dead and decomposing, potentially creating more nitrogen stress for the warm-season crop following it.
So if you were to grow a cover crop but kill it before its rapid stage of growth, it might do a lot of other good things like protect the soil from erosion, but it won�t really balance the soil bank account of nitrogen, because it�s not going to take up much nitrogen during the time of year when microbes are releasing nitrogen; anything that hits the rapid stage of growth increasingly will balance the soil bank account, but once the cover crop starts to flower, any additional benefits tend to decline.
While this all makes the management of cover crops sound challenging (and they can be), the reality is that many farmers use cover crops, and use them very well.
�The cover crop thing to me has been a very interesting aspect of agriculture because not only does it serve as ground cover, it absorbs nutrients especially if you�re putting manure down, but it also is a forage source. If you�re in the livestock business, there�s a tremendous amount of guys who are taking corn silage off, planting some cereal rye, and they say, �I�m going to save that field for spring when I�m calving my cows so I can put my cows and calves out there�, there�s no place better to put a cow and new calf than a 12 inch high field of cereal rye where she�s got fresh grass and a clean environment. Guys that do it- I haven�t heard any downside to it. They�re going to do it again the following year.�
This is Tom Pyfferroen, a farmer I�ve known for years whose constant tinkering with his production system I admire. For years, most of the acres he farms have been no-tilled and cover cropped, both things that can impact the soil bank account for the corn and soybean cash crops he grows. I was curious to see what observations he�s made about the impacts of cover crops, in particular, on how his soil behaves and how he manages nitrogen fertilizers.
�The term cover crop is always kind of an interesting term because, as long as I�ve been farming and for decades before that, a lot of people had small grains, and they always planted clover or alfalfa or some kind of a forage crop behind it. They didn�t always call it a cover crop, but I can remember the years when guys could plant oats, and if they didn�t have livestock, or they had a piece of ground that was maybe giving them some challenges, maybe it was a long ways from the barn, they�d plant sweet clover in it, and they�d plow that under as what they call a green manure cover crop�
But in today�s world of cover crops, I�d say we probably started in 2013, the year we had a lot of prevent plant. That was probably my first experience with annual ryegrass, turnips, and radish, and kale, and that type of language. And now it�s become kind of a norm, that list, every year it�s longer for different things people are using for a cover crop.�
2013 was a year that caused a lot of heartache for a lot of farmers in southern Minnesota. Coming off a drought the year before, the spring of 2013 brought with it almost nonstop rain and some late snowstorms, which kept the ground too wet for planting equipment to run across it until it was too late to grow corn, in particular, and still have a chance at decent crop yields. As a result, many farmers tried planting different things, including plants that are otherwise often used as cover crops.
�Once it started to rain in that fall of 2012, it never quit. It kept right on going into �13. That was kind of before we started doing any cover crops, and we had some full-width tillage out there, deep ripping and that sort of stuff, that stuff was just like snot walking on it. You couldn�t do anything with it�
As we know cover crops today, 2013 was my groundbreaking year on it. We got a lot of experience, because we had about 600 acres that we planted�
I think since we�ve moved to more no-till, if we had another 2013, we could probably get on a lot of that ground and do a pretty decent job without too much trouble at all. I think cover crops will carry you, but I think we�ve improved our water infiltration enough where that stuff is going down through the soil.�
�Speaking of water. Right now we�re in a drought year that�s showing no signs of letting up yet. What are your thoughts about the value and challenges of a high-residue system like a cover cropped one in a year like this?�
��you know, you have to be cautious in a year like this- and I had some experience this year- if you let your cover crop go too long, or you spray it and it doesn�t kill it, it�s going to dry out the soil. So you have to be cognizant of that- it�s going to happen to you every once in a while, that�s why I always tell people try and fail but never fail to try��
�I think if you�ve got the higher residue cover crop situation, and you got it terminated, and a little bit of rainfall so that stuff is laid down on the ground, I think you�re going to see the ground is probably going to be cooler under that stuff. We know it�s going to be more moist. And if it�s moist and it�s cooler, you�re going to have a field with less evaporation vs. a field that�s black. And I think you�d probably be pleasantly surprised at how much farther into the summer months you could go, comparing a no-till field that�s been cover cropped 2,3,4 years and a field beside it that�s been conventional tillage, I�d be willing to bet that you�re going to show stress a lot later in the season with that no-till field than you are with that full-width tillage field.�
�Yeah, so do you have kind of a typical cover cropping system that you like or are you always kind of tweaking it?�
�I�d say we�re always kind of tweaking it. Right now, with the last couple years, we started doing a little interseeding.�
Within the cover cropping community, you encounter terms like interseeding or planting green all the time, both of which refer to when a cover crop is planted or killed. In Minnesota, not exactly known for its mild climate, a cover crop planted in the fall after corn or soybeans are harvested might not have enough time to germinate and grow at all before the ground freezes, so anything a farmer can do to get plants in the ground earlier can make a big difference to how much of a cover crop he or she can grow, which will also impact how well that cover can balance the soil bank account. Interseeding, seeding the cover crop into a living stand of corn or soybeans so that it has already germinated by the time the corn or soybeans have been harvested is one way to do this. Planting green, which involves planting soybeans or corn into a field where a cover crop is still growing in the spring, allows the cover to grow longer and get larger in the spring, again, making it more useful in balancing the soil bank account of nitrogen.
�As far as nitrogen management goes, you know, I�m not sure we have a really good handle on how much nitrogen a cover crop will sequester, behind soybeans or behind a manure application. We�ve probably tallied our nitrogen applications for corn onto soybeans, we normally were probably running that 140 to 150 area, we�ve seen some 110s and 120s but it hasn�t hurt yield. Our yields have stayed up there. Is that from the mineralization of the cover crop at the time when the corn needs it most during that grain-filling period?��
�The other thing is, how much of the nitrogen that we put on does the plant have access to until we lose it, before it goes through the soil profile in a heavy rain environment or something like that that we never captured? I think there�s a lot of that. So with the cover crops and the roots they have on them, if we continue to build organic matter and carbon, we can probably trim that nitrogen use significantly yet. But where to, I guess, only time will tell.�
_______________________________________________________
Managing the soil bank account, the storage and release of nitrogen from soil organic matter, is a delicate balance. Too little release of nitrogen from organic matter means that crops have poorer yields and a higher reliance on nitrogen fertilizers; too much, and you risk depleting the soil organic matter that is the source of that nitrogen fertility, I�m often reminded when I see soils in different parts of the country that the incredibly rich supply of organic matter in the soils of the Upper Midwest is a fluke of geology and climate- that without the retreat of glaciers creating a landscape that was naturally wet and cool and led to a buildup of organic matter and nitrogen in soils, we could�ve just as easily been Kansas or southern Indiana, places where there�s much less organic matter in the soils and a greater need for nitrogen fertilizers. We are the beneficiaries of geography.
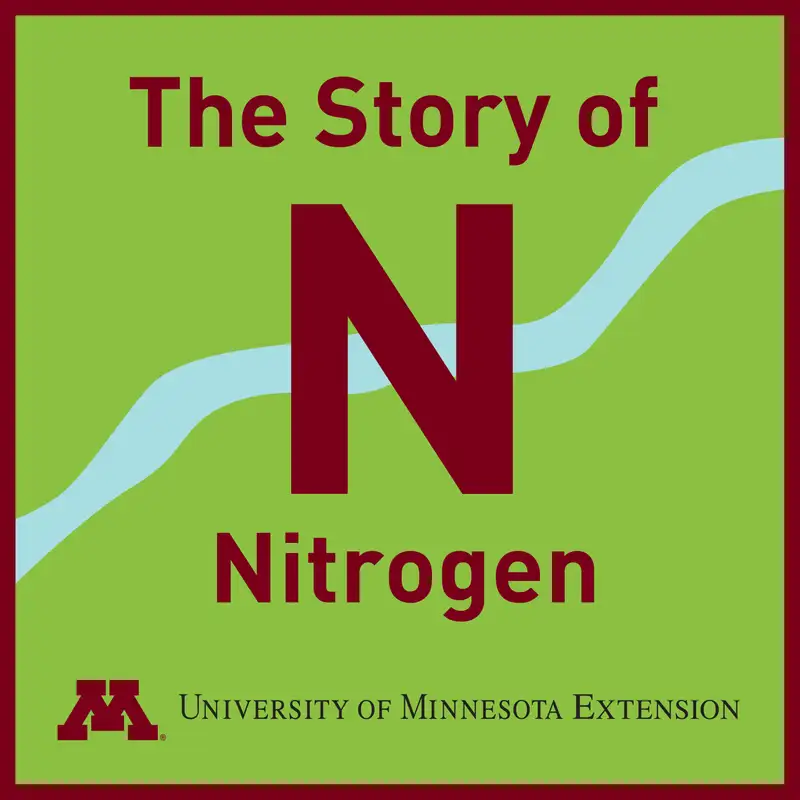