Episode 3- Winners and Losers
�Episode 3: Winners and losers�
�When did that extended diapause start to happen?�
�Well, it's probably always been here at a lower percentage. But in the 90s, in the early 90s, we started to see more of it...
guys could see it in more fields. It's just a simple math thing. You know, if you've got 100 rootworm beetles in a field, they're not going to do enough damage to notice, but if you got millions in the field, those populations are higher, that's when you're going to see the damage expressed.�
This is Bruce Potter, an integrated pest management specialist at the University of Minnesota. He�s telling me an interesting story of an insect that�s learned to adapt to how we manage our land with a change in its behavior. This insect is the northern corn rootworm, which, in response to human behavior, first learned to feed on corn, and has since learned how to survive for a year without food.
The northern corn rootworm is a nondescript little green beetle whose impact on crop production was until recently, anything but small. Its banana-colored, grublike larva is a voracious pest of corn that starts devouring corn roots after it hatches in the spring. It can prune back these roots so severely that the plant can�t take up water efficiently and can topple over like a tree in a windstorm. Until the development of genetically modified corn that produced a toxin that was deadly to these specific beetles, it greatly reduced corn yields and was responsible for a lot of insecticide use. It�s also an insect that has developed a winning strategy for survival.
�Can you tell me a little bit about the northern corn rootworm?�
�There�s two species of corn rootworms that cause most of the concerns In Minnesota- there's a western Corn rootworm and a northern corn rootworm. Western corn rootworm�
that we think is came out of the tropics and adapted to corn as corn was grown in North America. And northern corn rootworm was probably always here feeding on some of the prairie grasses. Larvae feed on the roots of corn and a few other grass species, but by far corn is the best host for them. And that species is a little bit unique... �
�So what's the normal life cycle of corn rootworm?�
�Okay, well, we'll start with the chicken and the adults emerge in July. Usually they started emerging about the time you start to see corn tassels appear, at least that's the way it is in Southwest Minnesota. And those adults will be active in corn, they may feel a little bit on leaves, their preferred food is corn silks and pollen, they�ll feed for a while. They'll mate�
as the silks and pollen quality disappears in corn, they'll move out and forage in soybeans, they forage on weed pollen both outside and inside the field. But as far as we know, in Minnesota, those adults are going back to corn to lay eggs�
the eggs are laid in the soil at the base of plants and usually fairly close to a corn plant...
then in about June, eggs will start hatching and the larvae will go through three instars, pupate, and adults emerge and start the cycle over again.�
�And they do not feed in any capacity on soybeans.�
�The, well, larvae cannot-�
and that's how for years those species were managed fairly effectively. You can, if you have a corn field, rotate it to soybeans, the larvae would hatch in soybeans and starve to death. But then the northerns developed this trait to basically delay that hatch...
To understand how this works, it helps to spend some time driving around southern Minnesota. In a lot of places, you�ll see one of two different crops growing in any field: corn or soybeans. And it�s pretty typical that a field that�s growing corn this year, will probably be planted with soybeans next year. If you were a corn rootworm, you could make quite a living during that year corn was grown: all in one growing season, you�d emerge from your egg, feed on corn roots and pollen from the tassels, mate, lay eggs, and die. But if that field was planted to soybeans next year, your offspring would have nothing to eat when they emerged next spring. It�s not a good longterm strategy for surviving in a lot of the Midwest. But, what if you knew that you�d have a good corn crop to feed your offspring TWO years from now? Then, you might want to figure out a way to get some of your eggs to hatch in two years rather than one, to hedge your bets. That�s basically what the northern corn rootworm has done- about half of them will lay eggs that will hatch the following year, and half will lay eggs that hatch in two years.
�A pretty large percent of that population has adapted to a Corn soybean rotation by extending the time period between when the eggs are laid and when they hatch. So instead of hatching the next year, they're going to delay that hatch for over a year. And the trait is called extended diapause that those eggs diapause for more than a year in the soil.
And that's how that species has been able to get around the corn soybean rotation.�
We change our cropping systems, and the northern corn rootworm changes how long its eggs diapause, or basically hibernate, in the soil. Above all, it has learned that in order to survive, it has to be adaptable. It�s not the only thing to have adapted to changes in how we manage our land. There are also creatures that have adapted to how we manage nitrogen fertilizers in the soil.
----
My name is Greg Klinger and I'm an agronomist and educator at the University of Minnesota Extension.� Together with my friend and colleague Shane Bugeja, I've spent the last few months interviewing experts in agronomy, biology, nutrient management, and ecology, trying to understand the story of nitrogen, in the hopes of explaining the phenomena we see out in the field, woods, and water.� Join us as we explore the different facets of this complex issue.
Episode 3, Winners and Losers.
--------------
It�s hard to over-emphasize how much life and diversity there is in the soil under our feet. For instance, in a single pinch of soil, you could have 50,000 different types of bacteria, � a mile of winding fungal threads, 100 tiny, barely visible roundworms. And all the ways in which these creatures make their living is truly staggering. Looking for creatures that, like us, get their energy by eating the carbon in other lifeforms and breathing oxygen? They are a-dime-a-dozen in the soil. But what about creatures that eat iron instead of carbon? There will be some in that pinch of soil. And how about living things that can survive by breathing the uranium in nuclear waste? You�ll find them in that pinch of soil, too. There are almost no chemicals or elements on earth that can�t be used by SOMETHING, for it to eat, to breathe, to reproduce. Life is tenacious.
And except for carbon, nothing on earth is used in as many different ways as nitrogen. A biologist might wince to hear me reduce the complexities of life in the soil to this, but for the purposes of understanding nitrogen and how it comes into or is lost from the soil, I think you can break down all life in the soil into four categories: the nitrogen fixers, the nitrogen consumers, the nitrogen burners, and the nitrogen breathers.
We�ve already discussed the nitrogen FIXERS in the previous episode, the microorganisms that live either in the soil or inside the roots of certain plants. These microbes pull nitrogen out of the air and convert it into usable forms for plants, animals and other living things. Without them, all 22 quadrillion tons of nitrogen (yeah, that�s a real number) in the air of Planet Earth would stay there indefinitely, and the only nitrogen in living things would be a small amount created by the occasional lightning strike or volcanic eruption. Life on Earth would be greatly reduced, and we would probably not be here.
When talking about nitrogen consumers, nitrogen burners, and nitrogen breathers, it�s helpful to relate them to how human beings survive. As human beings, we consume food, and separate out the carbon, the nitrogen, the water and everything else in it to build our own bodies. We burn up some of that carbon (like sugar) to get the energy to fuel our bodies, we breathe oxygen, and we consume the nitrogen in our food in order to build proteins in our bodies. When thinking about how all the different microbes consume nitrogen, some get their nitrogen from proteins built by other organisms like we do, others slurp different forms out of the water, or off the soil particles around them. But no matter HOW they obtain their nitrogen, every living thing needs it in one form or another in order to survive.
Some (generally less than 5%) of microorganisms living in the soil are nitrogen BURNERS. They burn nitrogen for energy the way we burn sugars, the way a fire burns the carbon contained in wood. Nitrogen burners don�t cause nitrogen to disappear, but their use of it for energy converts it to a form called nitrate. In a later episode, we�ll talk about this form of nitrogen a great deal.
Lastly, the nitrogen BREATHERS. You can understand the way nitrogen breathers live if you�ve ever run a marathon or exercised really hard, and felt your muscles start to burn. The most efficient way to make energy for our bodies is to burn sugars and breathe oxygen, but sometimes, when our muscles are working so hard, they burn sugar so quickly that they run out of oxygen faster than the lungs and blood can bring fresh oxygen to the muscle. When this happens, our muscles start to switch over to a less-efficient process that doesn�t need oxygen. This is called lactic acid fermentation, which lets our muscles continue to work, but leads to muscle burning, and small wonder- it�s the chemical equivalent of making sauerkraut in your muscles. The important thing to keep in mind, though, is the cells in our bodies ONLY DO THIS WHEN THEY CAN�T FIND ENOUGH OXYGEN. Nitrogen breathers live a similar lifestyle. When there�s oxygen in the soil, they breathe oxygen, because it is the most energy-efficient substance to breathe. But when oxygen starts to disappear, they switch to breathing nitrate, which is the next best thing for energy, converting most of it back to the form of nitrogen gas that�s in the air, all around us, useless to life. And just like that, a precious resource some hard-working nitrogen fixer created is gone.
All of these groups of organisms affect how easily we build up, retain, or lose nitrogen from our soils, and all of these microbes are affected by how we manage our land. But two groups in particular, the nitrogen burners and nitrogen breathers, are much more important than the rest in impacting how easily nitrogen is lost, particularly where fertilizers are concerned. To fully comprehend why, you have to understand that there are two major forms of nitrogen in the soil that plants and other living things consume for their growth, ammonium-nitrogen, and nitrate-nitrogen, and the two differ in how they behave when the soil is really wet. Ammonium, which with few exceptions is the form of nitrogen that fertilizers and nitrogen released from soil organic matter are rapidly converted into, tightly adheres to the particles in soil regardless of whether the soil is wet or dry. It�s also what nitrogen burners use to get energy for growth. The product the nitrogen burners create from ammonium, nitrate, is very easily lost from soil because nitrate DOES move easily with water through the soil, whether that water is moving up into plant roots, down to the bedrock below the soil, or sideways to a stream or ditch, but ALSO because nitrogen breathers convert nitrate to nitrogen gas when they run out of oxygen (which happens when soils are wet for days at a time). Without nitrogen burners and nitrogen breathers, you�d see almost no losses of nitrate from soil either to air or groundwater. So when a farmer is looking at a field of pale, stunted corn after weeks of heavy rain, and trying to decide whether it�s worth making another application of nitrogen fertilizer, one of the calculations he or she is making, whether it�s expressed this way or not, is: how busy have those nitrogen burners and nitrogen breathers been?
�.
There�s a famous scene from the old show �I Love Lucy�, in which the main character, played by Lucille Ball, and her friend, Ethel, get jobs at a chocolate factory, where they�re supposed to wrap the chocolates coming down a conveyer belt in paper before they get packaged and shipped off. At first, they manage the task all right, but as the conveyer belt speeds up, they can�t keep up. Panicked, knowing their supervisor will fire them if she finds any chocolates that make it by them unwrapped, they start stuffing chocolates in their mouths, down their shirts, in their hats. It�s a funny image.
And it�s a good analogy for how nitrogen behaves in the soil as it changes from form to form, and why nitrogen can be so easily lost from soil under certain conditions. Think of the conveyer belt, in this case, as the action of the nitrogen burners, converting ammonium and other forms of nitrogen [chocolates] in the soil into nitrate. Along the way, plants and other living things are grabbing some of those chocolates, that nitrogen, and using it for their own growth, like Lucy and Ethel. When the hands are grabbing fast or the conveyor belt is moving slowly, not much chocolate makes it to the end of the line, meaning that not much nitrate is produced and what is produced can be taken up by plants, if they�re present and growing. But when the hands are grabbing slowly or the conveyor belt is moving rapidly, a lot of chocolate makes it to the end. In other words, when a lot of nitrate is produced, there�s often more nitrate available than any plants can use, and when this happens, the excess nitrate can be lost by the activity of nitrogen breathing microbes or by moving down through the soil until it�s deeper than plant roots grow. I wanted to ask an expert a little more about these nitrogen burning microbes and under what conditions they can speed up or slow down that nitrogen conveyor belt:
My name is Chelsea Carey and I live in California. I am the working lands research director and principal soil ecologist with a nonprofit called point blue conservation Alliance.
I reached out to Chelsea because she�d written an interesting paper about what happens to the populations of nitrogen-burning microbes in the soil (the ones that produce nitrate from other nitrogen forms, making it more available for loss from the soil) when you start increasing their supply of nitrogen through some sort of fertilization. I asked her about the nitrogen burners, which can be split into two main groups:
Some of them are something I think probably most people wouldn't be familiar with called archaea. And some are bacteria. Could you just kind of describe those different organisms?
Yeah, totally. So, um, our Yeah, so many people, I think, are familiar with bacteria. They're sort of a sister phylum in many ways as archaea, which is less lesser known, they are both microorganisms.
And they share some key traits�
There is evidence that the archaea and the bacteria have different physiologies. They have potentially, like different ecological preferences and even like, optimal conditions. And it's interesting, you know, when I think about, and maybe this is a little tangent, but because they're sort of out of sight, out of mind, it's hard to see this, I think it can, it can be quickly lost that all of these below ground organisms, even if they do the same function, they can have their preferences and, and sort of differences can be like, sort of as varied as you had with wildlife, right, above ground. And, you know, just like a, you know, organisms or wildlife above ground might prefer to eat shrubs versus grass, or they might prefer warmer, cooler conditions, you can have the same thing with organisms below ground and that can matter, right for like, who thrives where. So even within sort of this population of nitrogen burners, there are differences between the bacteria and archaea that appear to matter.
Can you give some examples?
The archaea can use ammonium, like much more efficiently, they have a higher affinity to it than bacteria. And this can allow them to do better under lower nitrogen conditions. Right? So and that can matter when we start to talk about things like soil pH and stuff. There's also like evidence that the archaea do not tolerate high levels of ammonium in the same way that bacteria can. So they actually can be inhibited when we put a whole bunch of fertilizer down, and archaea don't like it, where the bacteria can thrive.
You made a comparison to wildlife, which I think is always super helpful to just kind of like, give some sort of frame of reference to these things. And the way I always think of them in my head, I always think of them as like a sloth. They're just kind of there, they're not really doing a whole lot. They're just like, they don't need to eat much. They just kind of sit there.
The inability to respond to conditions i think is such an interesting concept. And like, the analogy, I really like your sloth analogy, the analogy that I often give as well is like, let's say, you know, I am somebody who is on a, you know, like, I am gluten free, I am vegan. And then if you put me if you put a whole spread, a Thanksgiving spread of this delicious meal that that has a bunch of gluten, that it has a bunch of sort of ingredients that I cannot or will not eat, than it does, I'm not going to respond to that I can't actually eat it, right, like I and so I think I want to think about why organisms might not respond to added substrates, right? That's one example that I'll give sometimes is like, just because it's there doesn't mean you can take advantage of it necessarily because of your physiology or your preferences.
Not to torture the metaphor too much, but if I think of the archaea as sloth-like, then the nitrogen-burning bacteria are some sort of cross between a rabbit, which can explode in numbers when there�s a lot of food available, and a wolverine, which is famous for being able to eat huge quantities of food in short periods of time. Both groups of microbes eat the same thing, but the sloth-like archaea are designed to eat fairly sparingly and burn as little energy as possible, while the wolverine-rabbit-bacteria are designed to eat as quickly as they can. So when they have a lot to feed on, because there�s a lot of nitrogen from fertilizer or from the soil itself, their populations expand and they leap into action�or at least that�s the way we�ve understood them to behave. By the way, while I might describe these microbes as different types of animals, if you were to zoom down to the microscopic level and observe these bacteria and archaea, they wouldn�t look much different: just a collection of little spheres or TicTac-shaped organisms clinging to the edges of particles in the soil- their differences aren�t on the surface but in how they behave.
Chelsea�s research looked at what happened to populations of these sloth-like archaea and wolverine-rabbit-bacteria across a wide range of ecosystems that included natural forests, grasslands and agricultural fields, and asked: what happens when you either A) start adding nitrogen fertilizers to the environment, or B) increase the amount of nitrogen fertilizers you�re applying to that landscape. Do these nitrogen burners increase in abundance, and is it the bacteria that increase more or is it the archaea?
Overall, the bacteria responded much more strongly to fertilization like across the board than archaea.
What are the details of that response? For example, if I were curious about, let�s say, what logging some forest would do to populations of grouse, there�d be a couple things I�d want to know. One would be, you know, if I suspect logging wil increase the grouse population in the woods, I�d want to know by how much- double it, triple it? I�d also want to know how frequently that would happen- if I logged 10 different areas of forest, how often would grouse populations increase? 50% of the time, 100%? Translating that to nitrogen burners, what�s the magnitude of their population increase and how frequently does it happen?
Mm hmm. Yeah, well, I can say the magnitude. So from my study, the magnitude of response for the archaea, their abundance increased with fertilization by 27%, on average, across studies included in my analysis, the bacteria responded much more dramatically. And the average abundance of the bacteria increased by 326% in response to fertilizer, sort of across all the studies.
This is critical for several reasons. First, it�s what you might expect: the type of microbes that increase with more nitrogen fertilizer are the same ones you�d expect to: the wolverine-rabbit bacteria that can very rapidly consume a lot of ammonium in their nitrogen burning process. It�s also important for another reason, because there are chemicals that can be applied to fields that slow the nitrogen-burning activities of bacteria, but seem to have inconsistent effects on archaea- if the archaea were more dominant in the nitrogen-burning process, this would be a problem, but because they aren�t, it isn�t.
If you were to look at like, just baseline conditions, for these populations in an agricultural field, like, for me, that's a corn or soybean field in the Midwest, and compare that to some sort of natural ecosystem, what would kind of be the differences in those populations, just like baseline without even having to think about the fertilizer component?
I think all else equal between two, you know, sort of a managed agricultural system and an unmanaged system, I might actually expected that maybe population sizes would be greater in the unmanaged system a little bit if you don't have water logging of your soils, if you have and, you know, you don't have disruption of the soil surface and tilling or any other sort of mechanical disturbances, but when you add on that layer of fertilizer addition, I think then you're going to start to see that yeah, your agricultural lands are going to have higher populations of these organisms because you're increasing that substrate supply�
-by which she means the amount of ammonium available as an energy source for the nitrogen burners. More fuel for the nitrogen burners means a larger population is selected for over time, and so their numbers tend to be greater in agricultural soils that receive plenty of fertilizer. But there�s another factor going on here, which is that their RELATIVE prominence goes way up in agricultural soils, for two reasons. The first is that, at least where row crops are involved, there are times of year when there are no plant roots competing with the nitrogen burners for nitrogen. The second is that agricultural soils tend to have less microbes overall than the forests and grasslands around them. Chelsea mentioned that where there�s a large population of microbes actively feeding and growing in the soil, the nitrogen burners, which she calls by their more scientific name, nitrifiers, aren�t very good at accessing the ammonium in the soil:
�in many cases, we think of nitrifiers as not great competitors, actually.
Think back to the I Love Lucy chocolate factory conveyor belt. You have nitrogen (chocolates) moving from one form (ammonium) to another (nitrate) over time through the action of the nitrogen burners. Along that conveyor belt, though, plant roots or other microbes in the soil (Lucy and Ethel) are taking some of that nitrogen, allowing less nitrogen to be converted to nitrate. But if you have fewer of this broader group of microbes competing for that nitrogen or living roots to take it up, as is generally the case in agricultural systems, it�s like less hands grabbing chocolates off the belt- more ends up being converted to nitrate. And, at least in row crop settings, you often have the nitrogen burners moving that conveyor belt along at times when there are no plant roots there to pull off any of that nitrogen. The lack of competition means there�s little to keep the nitrogen burners in check; and they�ll work away on any ammonium they can find in the soil, increasing the possibility of nitrogen losses. This helps explain why nitrogen burners are such a thorn in the side of farmers everywhere, and why there�s a huge agricultural industry built around chemicals that can slow the activity of these microbes in soils.
If you�re walking through the woods early in the spring as the earth is thawing, oftentimes you�ll see the hyacinths, ramps, spring beauties and other wildflowers poking their heads through the snow, breathing, taking up nitrogen- pulling some of those chocolates off the conveyer belt. Walking through a field at the same time of year, mostly what you see is the cut up stalks of last year�s crop- there�s nothing growing there to compete with those nitrogen burners, who, while generally pretty sluggish until mid-April or so, become increasingly active as spring wears on. There usually isn�t much competition for the nitrogen burners until June, when the planted corn, soybean, whatever, crop has emerged and started to grow like crazy. All summer long, those crops suck up nitrogen like there�s no tomorrow, but if you were to walk through the field and woods again at the end of September, the crop field would be withered, brown and dead, waiting to be harvested, while there would still be plenty of green ferns, shrubs, and wildflowers to be found in the woods. And the nitrogen burners would still be working on any ammonium in the soil, moving it along that conveyor belt until late fall, when the soil was again cold, making it easier for nitrogen to be lost from the soil.
At the same time, the conveyor belt is chugging along faster in a row crop setting, because the populations of nitrogen burners out there have adapted over time to more quickly convert all that nitrogen over to nitrate, like the wolverine-rabbit crosses they are. A lot of innovation in agricultural products and practices has been spawned as a way to alter the dynamics of this conveyor belt and minimize those nitrogen losses. These include applying nitrification inhibitors, chemicals that slow down the activity of the nitrogen burners for a while, or applying nitrogen fertilizers at a time of year when there�s an actively growing crop to compete with the nitrogen burners. Much like the northern corn rootworm represents adaptation to a landscape of corn and soybeans, these types of management practices represent adaptation to a landscape where nitrogen burners thrive.
-----
What if there were a way for nitrate to be removed, without it being lost to the air by the actions of the nitrogen breathers, or being pulled into groundwater with percolating water after heavy rainfalls? This is something that happens in soils all over the world, but we didn�t realize it for the longest time, because we just weren�t looking for it.
Let�s go back to that �I Love Lucy� conveyor belt, where fertilizer nitrogen is being converted to nitrate nitrogen. When soil scientists measure this process, which helps them understand the potential for nitrate loss from the soil, they generally measure the �chocolates� (ammonium-nitrogen) as they are placed on the beginning of the conveyor belt, and the chocolates coming off the end of the conveyor (nitrate nitrogen). The challenge is that there are some recently discovered creatures that can use nitrogen in a way that runs the �I Love Lucy� conveyor belt in reverse, converting nitrate back to fertilizer forms like ammonium. If you were just measuring the ammonium at the beginning and the nitrate at the end of the conveyor belt, and how they�re changing over time, you would miss this internal recycling of nitrogen entirely- and that�s what happened with an unusual type of nitrogen breathing, called Dissimilatory Nitrate Reduction to Ammonium, or as I�ll call it because I don�t get paid by the syllable, DNRA. DNRA, by running the conveyor belt in reverse, keeps nitrogen in the soil where �regular� nitrogen breathing removes it.
I talked with Sada Egenreither, a researcher who studies DNRA, to learn more.
�When was this kind of, let's say, lifestyle of nitrogen breathing, where it's this short circuiting of the system, when was it first discovered or kind of realized that it existed?
I want to say it was late 70s, early 80s, definitely, by the 80s...
And it was actually originally discovered in I want to say it was in cattle rumen, it was in some like really specific context. And then for a long time, and this is why it hasn't been studied in soils until very recently. It was discovered in cattle rumen. And then, you know, that was kind of expanded to things like anaerobic digesters, or you know, swamp sediments, or things that are completely anaerobic 100% of the time�
And so, at what point did people start realizing it might exist in environments beyond these very anaerobic situations?
So that happened within the last 10 years or so�
So it wasn't until people happen to accidentally kind of measure it just as a suite of other things they were measuring were like, Oh, that's weird. I don't know why that's happening in this regular soil. This isn't, you know, an anoxic sediment or so forth. And kind of around the same time, as with next generation sequencing technology for DNA sequencing started becoming really accessible. People were just going crazy, and, you know, sequencing DNA from soil like crazy. And, you know, somebody just notice oh, hey, there's a bunch of DNA for this one weird process that shouldn't be happening in aerobic soils, but apparently is, and it's just out here and environment in all kinds of places, it�s in grasslands, and forests, it's in ag fields, you know, it's out here. And like, microbes are not sentimentalists, like, they don't keep things in their genomes, just for fun, like, they're being selected for a reason. So they're using it. So that's when people start thinking, oh, maybe we should investigate this�
And investigate they have. For the last 5 years, Sada has been looking at how these organisms live in agricultural soils, an effort that could have both economic and environmental benefits. Because if that conveyer belt isn�t reversed and nitrate begins to accumulate in soils, it can leak out of the field with water, or into the air after nitrogen breathers get ahold of it. In really wet years, which seem to be more and more common in the Midwest, I�ve seen losses of nitrate that exceed 100 lbs an acre, a BIG financial hit to a farmer, with some negative effects on our water and air quality.
DNRA and �regular� nitrogen breathing actually start out fairly similarly, as they convert nitRATE-nitrogen to a form called nitRITE, a form that doesn�t exist long in soils for reasons I�ll explain. But then, the two processes diverge- most of that nitrite becomes nitrogen gas lost to the air with �regular� nitrogen breathing, and most of it returns to ammonium with DNRA.
�So that first step is actually shared by the two of them. Where they actually differ is how they handle the nitrite. And so denitrification obviously converts that to some sort of a gas product that is lost, and then DNRA converts that back to ammonium�
� for the longest time we thought that was just, you know, a microbe, or some groups of microbes would have all the machinery to do the whole end to end process. And that's how it worked. But within the past, you know, five to 10 years, we've started discovering that actually, it's very modular, and it may work more like an assembly line where they're like, living near each other- one does step one, and they kick it over to the next microbe who does step two, and then maybe they kick it over to somebody else who does Step three, and there are many, many steps in that process�
Yeah. So maybe, what I'm curious about is why, from the microbe�s perspective, to the extent that we can understand that, why would it go in one direction or the other? What's the advantage?
Right. Sure. So for the longest time, and this is another reason why it was disregarded was that, you know, if you have nitrite, and it's gonna go one of the two directions for DNRA or denitrification, this is like a basic chemistry standpoint, like basic high school chemistry, denitrification produces more energy when you do it�
Generally speaking, denitrification should quote unquote, always �win� over DNRA under normal circumstances. So generally speaking, you know, microbes are doing denitrification to get energy to live right, like, oh, there's no oxygen, I guess we'll just breathe this instead, and this is how we'll make our living. DNRA on other hand, there's something weird going on with it, because it does not generate as much energy, a lot of the microbes that can do DNRA can also do denitrification, like they have a choice and have chosen DNRA for some reason.
Why would they make that choice?
This is where it starts to get into the more speculative area where like, we're not there yet, but it seems that, you know, all signs are pointing towards a toxicity avoidance mechanism, because nitrite is extremely toxic.
If you�ve ever looked at the ingredients on a package of ham or other processed meat, you�ll often see sodium nitrite listed. The reason this nitrite is added is because it�s harmful to microbes: it will prevent them from growing on the meat for a long time. For this same reason, a buildup in nitrite in the soil will start killing microbes unless they do something to keep that nitrite in check. Which, perhaps, is why they do DNRA, even when it doesn�t give them as much energy as �normal� nitrogen breathing does. DNRA is a direct competitor with nitrogen breathing. Both use nitrate as the starting product, often within the same organism, kind of like the competition between breathing oxygen or lactic acid in the human body when it�s active. And this competition only happens when the soil is really wet and has lost its oxygen. But DNRA has a leg up over nitrogen breathing, because it can start when the soil is drier.
-----
Imagine being out in your backyard garden or a farm field during a dry spell, when the dirt is bone dry and powdery, hard as a rock and almost impossible to dig. If you were able to zoom in to what was happening at the microscopic level, you�d see that, contrary to appearances, there was still water in the soil. But most of the channels, cracks and pores in the soil would be filled with air- water would just exist as thin films along the edges of larger channels and within the smallest soil pores. Most of the microbes in the soil, unless they were lucky enough to be living in those water films, would be withered and shrunken, dried up- not necessarily dead, but in a state of suspended animation, waiting for the rain.
A rain comes, one of those big soakers that lasts for days. Channels in the soil start to refill with water, and all the microbes that have been lying dormant for weeks wake up as they rehydrate. There�s an explosion of activity, growth and reproduction comparable to what you might see wildflowers do on the forest floor in the spring. As all these microbes become active, they start to breathe oxygen faster than it can be replenished from the air above the soil and oxygen levels start to drop. As they drop a little bit (conditions you might see when the soil looks moist but not sopping wet) some microbes start this �assembly line� process of nitrogen breathing, transforming niTRATE into the highly toxic niTRITE. But at this point, there�s still too much oxygen in the soil for the rest of the nitrogen breathing assembly line to proceed and convert this nitrite to nitrogen gas. Whether it�s to keep toxic nitrite from killing them, to get some energy for growth and reproduction, or for some other reason we simply don�t understand, some microbes start that DNRA breathing process, reversing that conveyor belt, helping to keep nitrogen losses from the soil to a minimum.
The rain keeps coming, and soon all the channels and pores in the soil fill up with water. Oxygen levels plummet (because oxygen moves very slowly through water compared to how it moves through air). At this point (which you might observe as soil that glistens with water, or is super muddy, or starts ponding pools of water on its surface), the rest of the nitrogen breathing assembly line can proceed. And a lot of microbes have to make a choice: they can finish out the assembly line by the normal nitrogen breathing process, which will lead to that nitrogen leaving the soil as a gas, or by using the DNRA process. The question is, what makes them choose one way or the other, and can we as land managers tip the scales in favor of DNRA? And while nitrogen breathing generally has the upper hand when the soil is this wet, it seems that a couple key things CAN tip the scales towards DNRA.
One of those things is a higher amount of carbon (essentially, dead and decomposing plant matter) in the soil, which, for reasons that are complicated and not worth getting into here, helps DNRA produce more energy for a microbe than regular nitrogen breathing.
�Only in that specific circumstance where you have a lot of carbon relative to nitrate would we expect to see DNRA outcompete denitrification and that's been that's like solid science that's been established really, really well in anaerobic environments�
So if you have a lot of nitrate in the soil, but not a lot of carbon, and the ground gets really wet, the scales will tip towards nitrogen breathing. And if you have a lot of carbon but not much nitrate, they tip towards DNRA. But there are still a lot of questions to be answered here: Is it just the AMOUNT of organic matter, or carbon, in the soil that matters? Or will different forms of carbon affect how favorable DNRA is- will the fresh sugars that drip out the ends of healthy plant roots be better than the cornstalks decomposing on the ground surface, or will the types of carbon contained in newly injected hog manure be most effective? There are some tantalizing clues to these questions that suggest that TYPE of carbon does matter, but so far, nothing conclusive. There are ways to increase the amount of carbon you have in your soil, but this change happens only slowly; the other key factor that can tip the scales in favor of DNRA is, perhaps, easier to manage.
---
Every fall, combines and grain carts roll their way across the Midwest, chopping up and spitting out the sun-bleached stalks of corn and soybean plants while separating out the beans and corn kernels themselves. As they rumble across the fields, a tremendous amount of pressure, sometimes greater than 75,000 pounds, is transmitted from the machine through each vehicle axle down through the tires and into the soil itself. This pressure, especially if it happens when the soil is wet, is incredibly effective at crushing soil particles together, making the larger pores and channels between the particles smaller and smaller. This has a tremendous impact on the ability of the soil to store and move water and air, because large pores in the soil are the highways on which water and air both move. Without large pores between soil particles, air can�t easily enter the soil, and that means oxygen can�t either.
Farmers and agronomists have known for ages that this compacted soil with its weakened ability to take in oxygen can really reduce crop yields, creating plants with shallow growing, flattened roots that will only explore small areas of the soil. It�s also been known for ages that plants living in these compacted soils are very inefficient in taking up nitrogen, the assumption being that because they have a smaller root system, they can�t access much nitrogen from the soil, and that less ability for air to get into the soil means the nitrogen breathers are more active. It is possible that there is more to this story, though.
Think about the competition between DNRA microbes and the other nitrogen breathers- one group retains nitrogen in the soil by reversing the nitrogen conveyer belt, while the other group irreversibly removes nitrogen from the soil by releasing it as a gas. The DNRA microbes are the �only game in town� when there�s still some air and oxygen in the soil pores, which happens a lot more often in soil that hasn�t been compacted. In compacted soil, which lacks those large pores that can move oxygen quickly, even a little bit of soil moisture pushes the ground into the very low oxygen zone that allows regular nitrogen breathing to occur. Compaction starts to favor nitrogen breathing over DNRA breathing, and the result is less nitrogen recycling and more nitrogen loss. If we want to help retain nitrogen in the soil, one thing we can do is to try and create conditions that allow oxygen to easily move in and through the soil.
But are there other ways to tackle this problem, to control the populations of nitrogen breathers, nitrogen burners, and other microbes in the soil that sometimes create some side effects that are challenging to us? I�m reminded of the northern corn rootworm, the insect pest that adapted to a rotation of corn and soybean crops every other year by changing how long it took to hatch. This pest is still largely controlled, because corn has been genetically engineered to produce a toxin that specifically kills these beetles. In a similar vein, can we target specific microbes in the soil?
---
�So just in general, how diverse are these microbes that have this kind of pathway?
DNRA microbes are super, super diverse, you know, compared to like a, like what we call a phylogenetically narrow process, like nitrification, you know, it's a relatively small number of organisms that are capable of doing that, and you can usually identify them by their name. Conversely, you know, DNRA microbes, they breathe oxygen when it's there, you know, they're just going to do whatever, there's all kinds of them. They live all over the place�
�what you're suggesting, you know, we, we talked about like, okay, humans evolved from primates. And that's all like a pretty closely related thing, but then there are also previous divergences that would be like, you know, tigers or whatever, birds, etc, that are kind of increasingly less related to us than, say, a chimpanzee or a gorilla. And so it'd be kind of like what you're describing, it would be kind of like this- it would be if the gorilla and us and the tiger and the bird all can kind of eat the same thing, right? I mean, it's, it's not restricted�
And also the trees that are near us and the grass that we're sitting on, probably, that level of diverse community is a super diverse compare. I mean, you know, if you like, you know, yeah, like a banana can eat the same food as I do. Basically. It's kind of like the territory that we're getting into.
Yeah. Which, kind of, to me raises an important point, because at least in agriculture, and not specifically this process, there are certain processes related to nitrogen cycling that we often want to have some sort of control over. But kind of extending the analogy for something like this, it would be like trying to devise a chemical that could kill or inactivate plants, you know, certain plants and trees and us and gorillas and tigers and birds, that all can do the same thing, right? It's kind of-
Yeah, yeah. But not all plants and trees and birds, only ones that can do �the thing�. Yeah, they're like, you know, another cat that can't do the thing, it's fine. Yeah, so I mean, not all of them have it, only some.
Yeah, it just raises, you know, compared to something like herbicides or things like that, where they can kind of target specific things, it just creates a more daunting task.
Now, in the case of DNRA, we generally want to encourage rather than discourage the process in soils, and we�d want to discourage the regular nitrogen breathers. Sada mentioned the process of nitrification- nitrogen burning discussed before- as an example where the number of organisms involved are fairly small, and the types of microbes very closely related, at least among the bacteria. The similarity between these microbes and their relatively small abundance makes it easier to manage them chemically, like we do with nitrification inhibitors- in the example above, it would be like devising a chemical to shut down the activity of plants that were grasses while not having much impact on broadleaf plants, animals or people. To be clear, the chemicals used to control these microbes have some limitations: they only work TEMPORARILY (as in, for a few weeks), and it�s unclear how effective they are in shutting down the activity of nitrogen-burning archaea. But overall, it�s a relatively manageable task- apply the chemical with your fertilizer and it�ll do what it�s supposed to.
But the challenges are very different for chemical control of the microbes responsible for DNRA and nitrogen breathing. Consider how many microorganisms there are living in a pinch of soil: maybe 10 billion individual bacteria of perhaps 50,000 different types, not even counting the huge number of archaea and fungi. I�ve never tried to do the math to figure out how many pinches of dirt there are in an acre of land, but it�s definitely a number with a lot of zeros, which means more microbes in an acre of ground than I can comprehend; certainly more than I can imagine trying to control, and up to about 20% of those are capable of nitrogen breathing. And other than the fact that they can all breathe nitrogen, they are about as similar as grass and trees and birds and cats, as Sada said. Lastly, many of the nitrogen breathers can also �do� DNRA, which we want them to do: so, ultimately, anything that slows nitrogen breathing could also be detrimental to the DNRA process we want to encourage.
----
Can we ever get to a point where we can truly manage these populations of nitrogen-impacting microbes in the soil, to actively create winners and losers among the soil microbes that can help us retain, not lose, nitrogen in our soils? There are reasons for optimism; there are reasons for skepticism, as well. What�s clear, though, is that if we want to limit the impact of nitrogen burners in the soil, we need to be thinking not just about chemical control but about how to make them less competitive with other microbes and plant roots for nitrogen. And if we want to increase the ability for DNRA microbes to compete with other nitrogen breathers and help reverse the nitrogen conveyor belt, we need to be thinking about how we can increase the ability of our soils to move water and air so they don�t lose oxygen as easily, and about how we can increase the amount of carbon in our soils. We�ll be discussing just that in the next episode, as we talk about all the ways our management can release or store carbon or nitrogen in the place most of it is held in soil: organic matter.
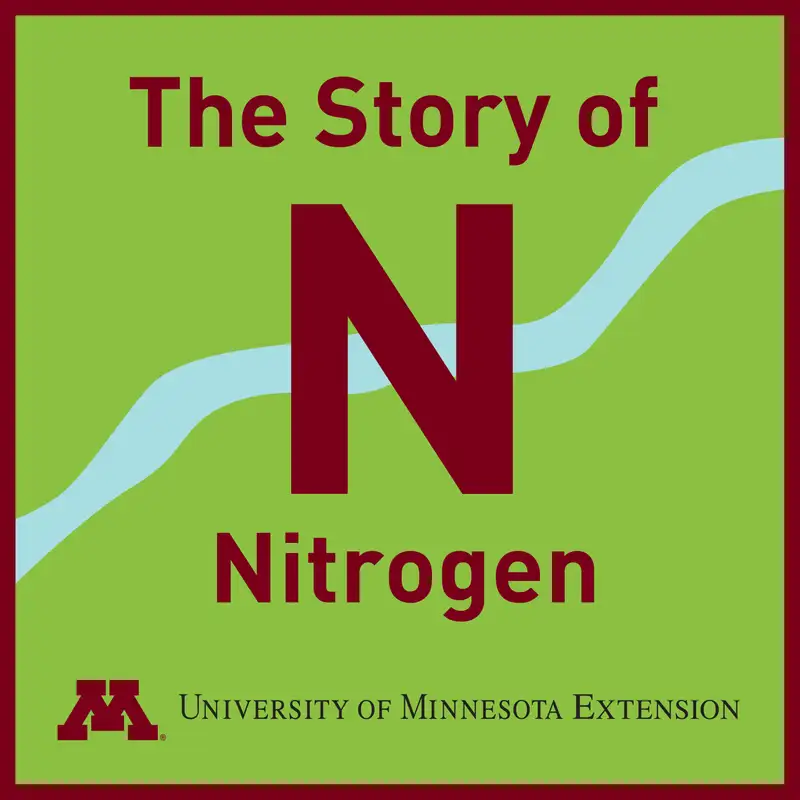