Episode 2- The Leaky Barrel, continued
The Leaky Barrel, continued.
Sometime early on in the existence of life on earth, some ancient ocean-dwelling bacteria-like creature, through the trial and error that defines all life, discovered a way to pull nitrogen out of the air, where it exists in near limitless quantities, and use it for its own growth. The molecular machinery it built to do this gave it a huge advantage over the other organisms around it, which struggled to scavenge scarce nitrogen from the ocean water they lived in. Many scientists think this development, which we call nitrogen fixation, was a key step in order for life to expand and flourish, and the organisms capable of producing this machinery were richly rewarded for it, passing it on to a variety of descendants that still exist today, when so many other creatures became evolutionary dead ends.
Fast forward to the Gulf of Mexico today, and you�ll find these types of nitrogen-fixing organisms exist in great numbers. In fact, you�ll find these organisms in just about any environment. They live in the cracks and pores of soil, floating in water, even inside other living things- algae, the guts of sheep, or the roots of plants. They can pull useable nitrogen from the air in endless amounts, enough to satisfy all the demand for nitrogen by living things, and yet they clearly don�t do this. To use the analogy of Liebig�s Leaky Barrel from the previous episode, where the total amount of plant or algae growth is visualized as water in a wooden barrel whose total volume is constrained by a shortage of a specific nutrient, visualized as a wooden stave on the side of the barrel that�s shorter than the rest, nitrogen still limits growth even when it doesn�t have to. Because, for instance, if you add nitrogen to Gulf of Mexico water samples, as Nancy Rabalais, a marine scientist from Louisiana, described in the last episode, many of the microscopic organisms floating in the water- things like diatoms and cyanobacteria, many of which CAN fix nitrogen- prefer the human-added fertilizer form instead. The question is: why? And why can a place like the Gulf of Mexico change so much when it gets more nitrogen coming into it from the rivers that feed it?
----
My name is Greg Klinger and I'm an agronomist and educator at the University of Minnesota Extension.� Together with my friend and colleague Shane Bugeja, I've spent the last few months interviewing experts in agronomy, biology, nutrient management, and ecology, trying to understand the story of nitrogen, in the hopes of explaining the phenomena we see out in the fields, woods, and water.� Join us as we explore the different facets of this complex issue.
The Leaky Barrel, continued.
----
I have a distinct memory from childhood of challenging my dad to a race while at my grandparent�s house, although I couldn�t tell you WHY. Not surprisingly, this was during my teenage years, where everything was a potential competition and I thought I was faster, stronger, better at everything than I really was. For whatever unknowable reason that parents humor the whims of their kids, my dad accepted, and we walked over to a nearby trail through the woods and started our run. I was determined to not just win but to DOMINATE, and so for the entire length of the race, I sprinted as fast as I could, until my lungs burned, my head was pounding, and my legs were like lead. Needless to say, I won by a large margin- but paid for it by spending the next half an hour lying down, muscles burning and trying not to throw up�while everyone else sat down to a nice lunch.
The story behind those burning muscles and that nausea has a strange parallel to the story of why some environments like the Gulf of Mexico change so drastically when their nitrogen supply changes. Both are stories of what happens when the DEMAND for oxygen by living things outstrips the SUPPLY of oxygen.
Think of what�s happening in your body when you start running at an all-out sprint, preferably when you�re in some bizarre competition to prove you�re faster than someone 30 years your senior. All your muscle fibers start twitching, burning as much energy as they possibly can, which requires them to consume a lot of oxygen. At the same time, your lungs start working harder, pumping more air and oxygen into tiny sacs in your lungs. But oxygen has a lot of work to do before it can get to the muscles that need it so badly. First, it has to move from the air sacs in your lungs into your bloodstream. Then, the protein that colors your blood red, hemoglobin, has to bind the oxygen and carry it to your muscles. Finally, it must be unbound from the hemoglobin and spread into your muscle cells before it can finally move into the cell structures where oxygen is being consumed. That�s a long journey, and when you�re pushing your muscles to their limits, they often need more oxygen than they can get, and so they start consuming other things for fuel instead of oxygen, which leads to the buildup of lactic acid that causes muscle burning and nausea.
And we owe a lot to that hemoglobin- oxygen doesn�t naturally move quickly into a liquid like blood from the air in our lung. Hemoglobin speeds that way up- without it, we�d all have been long dead of suffocation. Similarly, oxygen doesn�t move quickly from the air into a body of water, which creates challenges for the fish, insects, bacteria and other creatures that need oxygen. Now, these oxygen-breathers in the water don�t burn it up as fast as a sprinting human body, but there are some characteristics of lakes and oceans that can make them susceptible to losing oxygen faster than they can replenish it. As we saw in the last episode, changes in the supply of things like nitrogen and silica create biological winners and losers, but because more nitrogen often ramps up biological productivity- the equivalent of a runner picking up their pace- it also can affect the availability of oxygen in the water. But only when combined with the right physical conditions.
I want to go back to an interview from the previous episode with Chris Filstrup, a lake scientist from northern Minnesota. He and I discussed a condition that emerges at least twice a year on many lakes in Minnesota, when the water warms up in the spring and cools down in the fall, the separation of water at different depths in a lake, called stratification.
�So as the water warms up, it becomes less dense. And eventually you get to the point where the density differences are so great between the surface mixed layer that the wind can mix up, and the bottom waters that don't mix that it creates this really tight layer of demarcation of the surface mix layer versus those bottom waters. And it's kind of interesting because it is a temperature difference and it's pretty distinct. So if you're ever out on a lake in the middle of summer, and you have a really shallow change or density layer there, you can actually jump off your boat. And you can feel that temperature difference once you plunge through what we call a thermocline. But it's basically that change in density of water and you get really cold and you come up and you're like, Oh my gosh, why is it so cold down there. And the reason it is is because you plunge through the upper mixed layer, you plunge through that density layer and you were in the bottom waters, which are much colder because they're not interacting with the atmosphere and they don't receive a lot of Sunlight that could actually warm up the water.�
�So you can almost think of those as being two different lakes stacked on one another, right? Because the the chemistry, the biology in that top layer would be different than the bottom layer.�
�Yes, that's a very good point. But the thing to think about as well is that what happens in one layer affects the other layer, right? So as you have high nutrients and high productivity in that surface mixed layer, which is in contact with the atmosphere, so it's drawing in things like nitrogen, oxygen, from that surface mix layer, things that are growing there are eventually going to settle to the bottom of that lake. And because that bottom part of the lake is disconnected from the atmosphere, it doesn't get replenished with things like oxygen and nitrogen until the lake turns over in the fall and the lake mixes from top to bottom. So the problem with that is things produced up here settle down here. And then they're consumed, which causes oxygen depletion in those bottom waters. So it's very true. They are almost like different lakes and have different cycles going on which are driven by presence of oxygen and other things. But they're also connected.�
This idea of oxygen being consumed in the depths of a lake is worth exploring more, because it happens for the same general reason our muscles switch from respiring oxygen to lactic acid when they�re working hard- oxygen can�t get there fast enough to replace the oxygen being used up. In a lake, the oxygen is being consumed as organisms breathe or respire, and they breathe faster when there�s more food for them, and there�s more food for them when more dead algae, cyanobacteria, and diatoms are raining down from the surface of the lake. In contrast to a human body, it�s not so much that oxygen is being burned up quickly, as that it takes a very long time for oxygen to diffuse from the air to the bottom of the lake. Think of trying to dissolve a spoonful of sugar into a glass of water. When you dump the sugar in, it stays put on the bottom. It will dissolve and slowly spread throughout the glass of water, but if you didn�t stir it and drank it right away, you�d drink pretty normal-tasting water until you got to the bottom, when it would start to be very sweet. Eventually, without stirring, the sugar would mix into the water and the whole thing would be sweet. But it gets there way faster when it gets stirred. Similarly, layers of water have to mix in order to keep oxygen throughout a lake.
A similar thing happens in the Gulf of Mexico for much of the year as Chris described for Minnesota lakes, except the main thing separating or stratifying the surface water from the deeper water is salt instead of temperature. Freshwater is lighter than salt water, and so the water coming out of the mouth of the Mississippi, which is mostly fresh, flows on top of the saltwater in the Gulf. It�s only wind that really effectively mixes those two layers, the fresh and the salt, that are almost like two separate bodies of water stacked one on top of the other. And wind, especially during the �dog days of summer� can be hard to come by. As a result, the Gulf of Mexico stays stratified for a lot of the year, especially during the summer months, and most or all of the oxygen in the deeper saltwater layer can be consumed, a phenomenon that people refer to variously as the �Dead Zone�, Gulf hypoxia, or hypoxic zone. Fish and other things that live in the Gulf�s waters or the sediments below it can�t survive without this oxygen, and so they either migrate to other parts of the Gulf, up to the surface where there is still oxygen, or they die. Here�s Nancy Rabalais discussing the Dead Zone:
�In the Gulf of Mexico, we get the low oxygen areas, the hypoxic areas, primarily in the summer and is it right that it kind of extends into the fall?�
�Yeah. I have found it in all months except January. Okay. But the winter months there's too much turnover the water with cold fronts and in the summer sometimes with tropical storms or hurricanes, but pretty frequent for extended periods beginning in April through September, sometimes October.�
�Okay. And is it just the breaking up of those stratified layers that causes it to stop?�
�Pretty much. Yeah. Or, in some areas, deeper water that has more oxygen will move into shallower waters and alleviate the low oxygen for a while, but then the currents will usually push that water back offshore.�
�And when does that happen or what conditions let that happen?�
�Well, when we have to winds from the south east. They're considered downwelling favorable, which means that the low oxygen water mass at the bottom will move further offshore and into deeper waters. When the wind is from the north�
It pushes water offshore and relieves the pressure on the bottom so that the low oxygen can move in shore. And that's when we have what are often called jubilees or fish kills on beaches.�
�Okay.�
�So it's, it's, you know, mostly, I mean, it's all kinds of things. It's the wind speed, the wind duration, the currents, the direction of the wind, the speed of the wind- Just all kinds of things .�
�So hypothetically, -you could have a lot- the same amount of nutrients and water moving down the Mississippi into the Gulf of Mexico in one year as the next but if you got, let's say, a strong hurricane season, one year, the next, the hypoxic area could be very different.�
�It can and it has been very different. That is temporary.�
�Okay.�
�Because there's still plenty of carbon in the bottom to lead to respiration once it becomes really stratified.�
�So that would be-when you say temporary, you could have like a hurricane blow through and in what, like days, weeks, months, you could redevelop the hypoxic-�
�Days. weeks, okay, you'll have low oxygen again.�
During much of the warm months, the hypoxic zone or Dead Zone is the baseline state of much of the Gulf, because the nutrient-rich water generates huge amounts of algae that eventually die, settle on the bottom, and are decomposed, consuming oxygen, and the typical state of the water is to be stratified, preventing fresh oxygen from getting into the bottom water. Only when atypical conditions are present- when a major drought slows the river to a trickle, so that there is no fresh water to cause stratification, when the winds change to certain directions or a hurricane blows in- does the Dead Zone break up. I asked Nancy how someone spending time out in the Gulf would know they�re in the dead zone.
�You cannot see low oxygen because it's at the bottom. But when we're out on our research cruises and fishermen will often see animals that typically live on the bottom are up in the surface waters swimming, such as some of the crabs, some of the shrimp, some eels that live in the mud will go up 60 feet and be at the surface. And the fact that these organisms are up there is because the fish have moved down to the area, and they're not being preyed upon, because there's not enough oxygen in the bottom waters to support healthy fish populations, those that depend on the bottom, you can catch a tuna, cobia you know, all kinds of other things in the upper water column, which is why I try not to call it a dead zone.�
�Okay, because for all intents and purposes, you would still see life in your immediate area, even though-�
�You would see life in the upper water column: we have underwater videos that show all the fish and then you start to lose fish and you get to the bottom and it's not- You can't see and there are no fish. There's no growth on the offshore oil platform at the bottom, and there's tons of barnacles at the surface.�
�Any other ways you can see the dead zone- area of low oxygen in the Gulf?�
� there's been some research done by Kevin Craig and his group that if you do aerial photography of where the shrimp fleet is, it matches perfectly with where the low oxygen is. In other words, they're always on the outside of the low oxygen area. �
�Hmm, that's really interesting.�
�Yeah, the shrimpers are not stupid. They know where to go.�
-------
Think back to the idea of your muscles burning lactic acid when they�re working hard and can�t get enough oxygen. If your muscles can fuel themselves with lactic acid, why bother even breathing oxygen? Why even HAVE lungs?...
Turns out, there�s a good reason, and it has to do with energy efficiency. Breathing oxygen produces more energy than just about anything else on Earth. Breathing lactic acid produces much less energy, so much less, in fact, that there are no large living things that can rely on it for long- they would, in a sense, starve to death. LARGE living things are always dependent on having lungs or gills to breathe oxygen over the long term.
But many SMALLER organisms like microbes, because it takes less energy to keep their bodies running, can consume things other than oxygen when they need to. There are, for instance, many microbes found all across Earth that will start consuming nitrogen when oxygen supplies get low. In the same way that we consume oxygen and release carbon dioxide when we breathe, they consume forms of nitrogen like nitrate and release nitrogen gas back into the water or air. It�s basically the reverse of nitrogen fixation- biologically useful nitrogen returns to a form that is unusable.
This leads us to a paradox that often exists, particularly in bodies of water, which is this: the nitrogen going out tends to come into equilibrium with the nitrogen coming in, so that it maintains a high demand for nitrogen. This is how it works in the Gulf of Mexico: the Mississippi flows into the Gulf, carrying with it nitrogen, phosphorus, and other nutrients that keep the diatoms and algae in the water happily growing. When these organisms die and sink to the bottom, bacteria consume them, along with oxygen. As the oxygen disappears, the bacteria shift to consuming nitrogen, until, as you get farther out into the Gulf and away from the mouth of the river, there�s very little nitrogen from the Mississippi left.
Now imagine you increased the nitrogen supply flowing into the Gulf from the Mississippi. You�d get more growth of diatoms and other algae, which means more dead algae settling to the bottom of the Gulf, more bacteria consuming oxygen as they decompose the algae, which means a larger area of oxygen-free water for longer periods of time (the Dead Zone), which means more bacteria shifting over to breathing nitrogen, which means more nitrogen being removed from the water-
it�s kind of like someone who, when they get a pay raise, just starts spending more money rather than saving. Yeah, there�s more money coming in, but at the end of the month, the bank account doesn�t look any different. The overall demand for nitrogen by living things is just as high.
And because that demand pressure is still there to shape and mold the community of living things in the Gulf, more and more nitrogen will continue to create biological winners and losers. More growth of algae, as well as a shift towards more toxic species, as discussed in the last episode, means more possibilities for fish or animals or people to get sick by being on the water or eating things that came out of it. More growth of algae COULD mean the ability to grow more fish, especially those that directly feed on algae, but this is counterbalanced by the Dead Zone that becomes larger, killing some fish and displacing the rest. When you talk to biologists, it�s often unclear as to which factor, MORE fish because of more biological productivity vs. LESS fish because of the Dead Zone, is more dominant. What is clear, though, is that all these changes affect the lives and livelihoods of all those who live or visit the Gulf of Mexico. Here�s one example:
��the brown shrimp is the most economically viable shrimp catch. And what happens is that they spawn earlier in the year the larvae go into the marshes and then the juveniles are trying to move offshore during the peak of the low oxygen in extent and severity. Those smaller shrimp get cordoned off near shore. And the shrimpers know that, and they can catch tons of them. But they're very small and they don't bring much money. Whereas any brown shrimp that are able to get further offshore grow to a larger size and they're worth much more money-�
But the size of the Dead Zone has a direct impact on how profitable it is to catch these shrimp. Since these larger shrimp are forced to the outside of the Dead Zone, farther offshore, (and the Dead Zone can be up to 7000 square miles in size, roughly the size of New Jersey), the amount of fuel it takes to get that far out and harvest these shrimp can be the difference between a profitable shrimp industry, or one in trouble.
-------
In saltwater, more nitrogen leads to more growth, but how does it work on land? Because soil is generally faster at replenishing its oxygen supply than bodies of water, and because soil tends to store large amounts of nitrogen in it to begin with, the results of more nitrogen on dry land are decidedly more mixed. If you were to look at all the research studies where scientists add nitrogen fertilizers to land systems- anything and everything from deserts to grasslands to forests to cornfields- and investigate how much and how fast plants grew in them, you�d observe an interesting thing. The most likely result in these studies is that more nitrogen equals more growth, but there are many times where more nitrogen really doesn�t affect growth at all, and sometimes where more nitrogen means LESS growth. This inconsistency is intriguing.
I was able to dive more into the details of when and why you can see these different outcomes on land by talking with Mary Beth Adams, a researcher with the United States Forest Service. For decades, Mary Beth has been conducting research in a unique place, the Fernow Experimental Forest in the Allegheny Highlands of West Virginia. This entire forest is a longterm study site on how an increase in nitrogen fertilization can impact the growth of forests. The Fernow forest is one of a number of places in the United States that�s in a condition referred to as �nitrogen saturation�, a situation where most added nitrogen stops being used by plants. Thinking back to Liebig�s leaky Barrel, this is a situation where nitrogen stops being the shortest stave on the barrel, and something else is instead, with consequences including a change in what types of plants thrive in the forest, and more losses of nitrogen to streams, lakes, and rivers.
So why do some forests get nitrogen-saturated while others don�t? Like in real estate, the answer is generally location, location, location. The Fernow forest sits on the top of the first high mountain ridge downwind of the Great Lakes to the northwest, and Gulf of Mexico far to the southwest. All the water vapor in the air coming from those bodies of water cools as it rises, and it condenses into rain that falls on that ridge- lots of it. The area is just shy of being a rainforest. And all that rain brings something else with it- a lot of nitrogen. Some of this comes from the fertilizers or manure we apply to farm fields and lawns, but most comes from a more unexpected source- the burning of fossil fuels from vehicles and power plants, which always contain small amounts of nitrogen. Here�s Mary Beth:
�There's still that legacy of 75 years of high nitrogen deposition. And that's it's coming from the Ohio or it has been coming. It did come from the Ohio River Valley and all those power plants right along the Ohio River Valley that were, you know, powering steel mills��
Now to be clear, even in an environment that gets a lot of nitrogen fertilizer in precipitation like the Fernow, this nitrogen fertilizer is much less than what you might see being applied to lawns or cropland. Something less than 10 lbs/acre/year vs. the 120 and more lbs/acre/year you might see on a cornfield, for example. This longterm fertilization from the atmosphere can still change the way the forest operates, though, for the simple reason that forests, being mostly composed of plants that live for multiple years and sometimes even for centuries, store and release nitrogen far differently from a field whose plants germinate, grow, and are harvested every single year, and they�ve been receiving this nitrogen fertilizer from the air for the better part of 100 years.
�If I was out walking in the woods there in Fernow, could I actually see- if I were observant- could I see nitrogen saturation? And what would it look like?�
�There might be subtle differences in species composition, particularly like the herb layer vegetation that could reflect Nitrogen saturation for instance, there might be areas where there is more blackberry or raspberry��
�One of the other things we know that High available nitrogen in the soil increases germination some tree and plant species and particularly pin cherry or fire cherry is one of those that which responds with a flush of germination to high nitrate levels in the soil but it also requires a lot of light. So if you have a disturbance and you have a lot of nitrogen there, you might see a lot of pin cherry. So it tends to be very patchy and it might not be obvious.�
In the 1980s, when scientists started to realize that nitrogen raining down from the sky was going to be a persistent issue in America, researchers at the Fernow started to do an experiment that was meant to give us all a glimpse into the future. The idea was, if we speed up this process of fertilization, if we start to add two years� worth of fertilizer to our forests over what they�re getting now, what happens to our forests? Will they grow faster or slower? Live longer or die younger? Will they be healthier or more riddled with diseases and pests? So they started taking a small plane up twice a year and applying nitrogen fertilizers to areas of the forest, and then monitoring what happened to the trees. And what they found was more nitrogen meant faster growth- but only for some trees, and only for a short window in time.
�we measure the growth of trees, generally by measuring diameter at breast height, you know, four and a half feet��
�and what we saw was a short term increase in diameter growth, right after or very soon after we started fertilizing��
�in some tree species, and particularly the yellow poplar, and black cherry, we saw an increase in diameter growth, which looked like a response to nitrogen fertilization, like we would see in loblolly pine or, you know, sweet gum, or anything else. You fertilize it, you get an increase in growth. But it's not a long term, it was not a long term increase in growth� �
�Black cherry and tulip poplar grow like gangbusters, you know, they're they're early successional, but not pioneer species, I guess, and so they grow real fast, take advantage of all the resources they got. And black cherry basically is is just a weed physiologically and it'll take up all the nitrogen, almost all the nitrogen you give it��
�but red maple and birch didn't, didn't show a response��
�The other thing we did was do some tree ring work, core some trees and look at the chronology from the tree rings. And we saw that increase in growth. And then later on, we saw a decrease in growth. And the decrease in growth was actually also related to the amount of calcium in the tree rings��
� �We got a short term increase to nitrogen, and then calcium became limiting. And so the growth slowed down.�
�Which is interesting because that calcium availability is also directly tied to nitrogen fertilizer, you know, they�re kind of inversely related to each other.�
�Right. Right.�
This idea requires a little more explanation, but basically comes down to this: both calcium and nitrogen generally have electrical charges on them; calcium is positively charged, while the main form of nitrogen that moves around in the soil, called nitrate, is negatively charged, and the two attract each other, held close by an irresistible force. If you�ve ever used a magnet to play with some iron filings, you might understand this concept. If you get the end of a magnet up against some metal shavings, you can drag those shavings basically wherever you want to with the magnet, because the magnet has either a positive or negative charge and the iron shavings have an opposite charge. The two attract each other, and so the shavings will follow the magnet wherever you drag it- the same goes for the calcium and the nitrate. Nitrate in the ground is also, however, attracted to water and will move wherever water does (much more on this later). So what often happens is that water moving down through the soil after a rain will pull nitrate with it, and that nitrate in turn pulls calcium along with it. Now, in a crop field, this isn�t as much of a problem because we can replace the calcium that�s lost this way by adding lime if we need to. But we�re not going out and adding lime to all our forests. That would require a lot of crop-duster type planes, a huge amount of lime, and an absolutely unbelievable budget to do on any large scale.
The way in which nitrogen affects calcium and the growth of plants adds a twist to Liebig�s Barrel- the idea that the growth of plants and algae, visualized as water in a wooden barrel, is limited by whatever nutrient, each visualized as a wooden stave on the side of that barrel, is available in the lowest relative supply, regardless of how much supply there is of other nutrients. In real life, one stave on the leaky barrel can also affect the height of another stave. In the Fernow, more nitrogen means less calcium and eventually less growth (raising one stave lowers another). There are other connections between staves, too- for instance, in crop fields, we often see that when there�s not a lot of the nutrient potassium, crops sometimes need more nitrogen than they otherwise would, and sometimes less, a confusing and hard-to-apply relationship. The more you study it, the more complicated Liebig�s Barrel gets, with real-world impacts. We just live with the consequences and try to manage them as best we can.
�So nitrogen sometimes added to growth� - short term. Does it ever- have you seen instances either in the Fernow or just elsewhere where it actually leads to a decline in growth?�
�There are there were some other nitrogen addition studies, one of them with pine I think and adding too much nitrogen�
there was, you know, a point above which it basically out-and-out killed the trees over the course of several years�
The trees were less cold tolerant and by just a few degrees and when you got those really cold snaps, you know those really low temperatures for a day or, you know, two in the winter, it would freeze or you know, the foliage it would turn red and and didn't photosynthesize that year, and if that happened more than a year or two, or if it happened more than a year, then the trees basically were without photosynthesis, and would starve to death...
And we're pretty sure it was nitrogen that was affecting it...
So I think that conifers are probably more susceptible�
you can mess with the physiology of a tree by adding too much nitrogen.�
A parallel situation can happen in pastures that get too much nitrogen too late in the fall in cold climates- the pasture grasses can actually become less cold tolerant and die during winter cold snaps, something you don�t notice until they fail to green up the next spring. But some plants are more susceptible to the negative effects of nitrogen than others. And a few plants will try and acquire all the nitrogen they can.
----
One of my favorite things to watch in the spring here in southeast Minnesota is the greening up of alfalfa in the field. You see it growing in narrow ribbons of land on many hillsides around here, and in the spring, its green is a nice contrast to the tan or gray of last year�s corn or soybean crop around it. Alfalfa is a type of plant know as a legume, a group of plants whose ancestors millions of years ago created one of the most powerful alliances of mutual benefit in the natural world when they started letting nitrogen-fixing bacteria live inside their roots. These bacteria pulled nitrogen gas out of the air surrounding plant roots in the soil, and transformed it into forms that they, plants, and other living things could use. In exchange for providing that nitrogen to their host plants, they received the sugars they needed to fuel their own survival from the plant, which can produce huge amounts of sugar every day from photosynthesis. This cooperative relationship between bacteria and plant allowed the legumes to become one of the most dominant type of plants in the world today, found in environments the world over- forests, grasslands, deserts. They also make up some of our most important food crops, like beans and peas, and feed crops for animals, like soybeans and alfalfa.
There are environments where legumes dominate, generally where the soil has been disturbed recently, by logging, wildfire or construction activities, for example. I can recall a hillside I drove by every day for years that was torn apart and rebuilt in order to build a highway on-ramp, and for years after that construction, the whole hillside was covered by nothing but vetch, a type of legume. Legumes can dominate these environments because these disturbances often remove a ton of nitrogen from the soil. And if a plant is starved for nitrogen, the ability to pull nitrogen out of the air becomes incredibly valuable. To get a sense of how substantial a source of nitrogen fixation can be for legumes and legume-like plants, take as an example the red alder, a nitrogen-fixing tree you can see growing across the Pacific Northwest, particularly after the land has been logged. Groves of these trees can gain 150 lbs/acre of nitrogen from the bacteria in their roots; a stand of alfalfa can gain a whopping 250 lbs/acre of nitrogen in this way. These might not seem like huge amounts, until you consider that the 250 lbs of nitrogen is about all the nitrogen you�d find in 10 giant round bales of alfalfa hay out drying on a hillside. And that 250 lbs/acre of nitrogen that alfalfa can fix is far in excess of what�s contained in a good corn crop. Being able to obtain more nitrogen is so valuable to a plant�s survival that even some plants that can�t fix nitrogen have developed their own novel strategies. For example, there�s a variety of corn, discovered recently in Mexico, that oozes a sort of gelatinous slime down its stalk- this slime slides down to the soil and specifically feeds bacteria that fix nitrogen, which the corn presumably can then use. Here�s Mary Beth talking about legumes in West Virginia forests:
�There are some forests that have a lot of nitrogen fixers in them. And black locust is one that we have here in the early stages of forest growth. It's not usually a very long lived tree in our forests, but it takes up- it creates a lot of nitrogen, puts it back into the soil.�
Legumes like black locust have leaves rich in nitrogen. Every fall, as those leaves turn yellow, drop down to the earth, and decompose on the forest floor, they release nitrogen that slowly accumulates and can be used by other trees and shrubs to help their own growth. So, in a sense, while the locust tree is trying to get more nitrogen for its own gain, it has the downstream effect of also creating a richer environment for other trees. We see the same thing happen when we plant a crop like corn after growing a legume like alfalfa- that corn needs much less fertilizer than it otherwise would, because the alfalfa has created a soil that�s rich in nitrogen that the corn can now use.
�Yeah, I always think of black locust as the fence tree, since you see it so often as a fencepost or in split-rail fencing, or just growing in sunny spots in old pastures. What�s the advantage for a legume tree like a locust to actually fix nitrogen?�
�If nitrogen is the nutrient that normally limits tree growth and that's, that's been our dogma for a long time, then the trees that can fix nitrogen- take it out of the air and convert into biomass- are the ones that are going to survive.�
�That makes sense. At the same time, I know that most trees in our forests aren�t legumes, and you mentioned that even locust trees don�t live long in the forest. So why is that- what�s the downside?�
�I guess it has other costs. You mentioned needing lots of sunlight. You know, I think that's part of it. At some point, the other trees over-shade them, overtop them and shade them out and they can't produce enough photosynthate to continue to fix nitrogen and grow.�
There�s a reason why organisms that can fix nitrogen might not fix enough to meet their own requirements or the desires of the other plants around them- they don�t always get rewarded for their efforts ..and sugar that otherwise might be used in growing taller or making more seeds is instead fed to a microbe. . You can see the disadvantage of being a legume when you�re walking through a prairie or old hayfield. The leaves of the legume plants in these environments- things like alfalfa, peas, clovers, wild indigo, leadplant- tend to be round or in clusters, and they tend to grow out horizontally more than up, while non-legume plants like grasses tend to grow up way more than out. You can see also this the next time you�re driving around in the countryside and see how soybeans (a legume) planted in wide rows flop over, while corn (a grass) planted in wide rows grows up straight. And so as legumes add more and more nitrogen to the soil, grasses and other plants around them are able to grow taller and start to shade the legumes out. Walk through an alfalfa field that�s 4 or 5 years old, and you can see this happening before your very eyes, as the alfalfa starts to get replaced by orchardgrass or bromegrass.
The challenges associated with turning air into fertilizer is not limited to microbes and plants. Many farmers might still remember their fertilizer bill from 2008, when natural gas, crucial to making nitrogen fertilizers in factories, quadrupled in price. This cost, whether it�s the cost of making nitrogen in a factory or in bacteria, will always impose limits to how much nitrogen is being produced and used. But there�s another thing to consider when trying to understand why nitrogen fixers do not generate enough nitrogen to fully remove nitrogen limitations from the environment, which is that legume plants don�t live in a vacuum- they inhabit a world filled with animals that eat plants, animals that ALSO need a nitrogen-rich diet to survive.
�There are a lot of studies in different forests that say that when there�s more nitrogen in plants, their leaves usually become more palatable, and so they get more feeding by things like insects or slugs, maybe even animals. The idea is that this is one potential downside to having more nitrogen, one downside to being a legume. Have you seen that sort of thing in the Fernow forest?�
�It's not something that I'm most familiar with, but I know from experience if you plant a tree seedling from a nursery in certain parts of our woods, the deer will find it and eat that one. It's, you know, they're pre-loaded with nutrients, not just nitrogen, but, you know, when they come out of the nursery, they're at their supposedly optimum nutrition...
you know, we put out an oak seedling- we were looking at seeing which grew faster, nursery seedlings or acorns and they would always find the nursery seedlings. Just they like, they know. They know, they smell different or something, I don't know.�
�I�m still curious about one thing. If trees will grow faster if you give them more nitrogen, why isn�t every tree in the forest become a legume, or at least why don�t the non-legume trees help the legumes growing around them? I mean, our trees have had millions of years to evolve being better at generating nitrogen.�
�well, there's a difference between optimum productivity and survival. You know, people can survive on very little amount of calories- most of us don't. And growth, you know, our optimum growth or health is, you know, a well rounded diet.�
�Okay, so some of the winners, I guess, of higher nitrogen are these cherries and the raspberries and blackberries and things like that. What about in the soil itself? You know, like microbes and things living in the soil? Are there any particular winners and losers from more nitrogen being cycled through the system?�
�I think we've seen that fertilization can often increase fungi at the expense of bacteria�
�and one of the things that we know is elevated nitrogen deposition over time acidifies the soil�
And so it can change soil pH which moves it more towards lower pH which favors fungi over different kinds of bacteria.�
...It�s a good reminder that every action we take involves putting our thumb on the scales, and that this creates new challenges for us to manage, new winners and losers. And these winners and losers extend from the largest trees to the tiniest bacteria, and everything in between. As we�ll explore in the next episode, EVEN WHEN we can control many of the effects of changes in nitrogen, for example, by counterbalancing the acidifying effect of nitrogen fertilizers on our crop fields by adding lime fertilizers, we�ve changed the winners and losers in the soil itself.
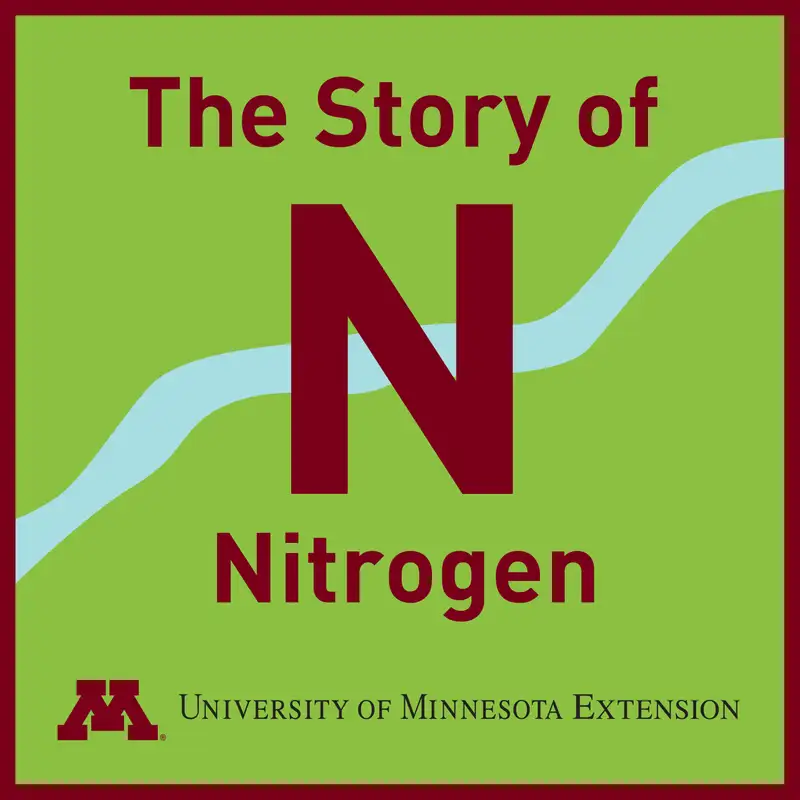